School Research Seminars
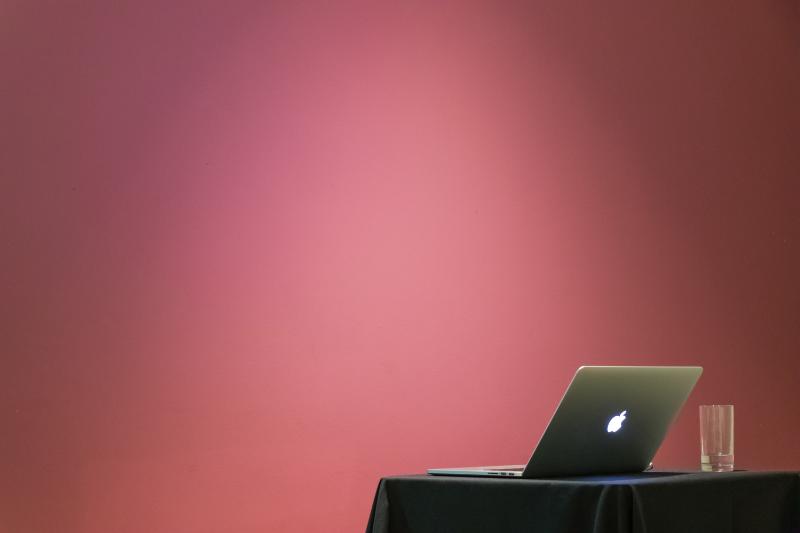
Each academic year, the School of Chemistry and Chemical Engineering hosts a diverse range of research academics from institutions across the globe, providing the opportunity for discussion, collaboration, and idea sharing in relation to cutting-edge research within the chemical sciences.
Upcoming School research seminars are detailed below - should you wish to attend one of the seminars, please contact our School Seminar Coordinator.
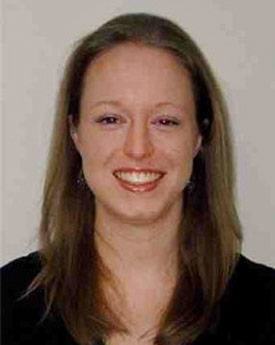
Converting simple starting materials into complex products using only a light source (synthetic photochemistry) is especially attractive to organic chemists, particularly from the point of view of green chemistry: waste is minimized, and light is readily available. In addition, photochemical routes often allow efficient access to complex frameworks (particularly to strained molecules and intermediates) that cannot be generated using ground-state chemistry.
In this seminar, Dr Coote's recent work on the synthesis and applications of bicyclic 1,2-diazetidines2 will be presented. Bicycles2 can be obtained in high yields from 1,2-dihydropyridazines1 simply upon irradiation at 350 nm, and are versatile synthetic intermediates that can be converted into a variety of different derivatives, including substituted 1,2-diazetidines, cyclobutanes, cyclobutenes and dienes (Figure 1).1,2,3 In addition, related work on similar systems will be discussed, including ongoing unpublished work.
Figure 1.
References
1. Britten, T. K.; Kemmitt, P. D.; Halcovitch, N. R.; Coote, S. C. Org. Lett. 2019, 22, 9232
2. Britten, T. K.; Akien, G. R.; Kemmitt, P. D.; Halcovitch, N. R.; Coote, S. C. Tetrahedron Lett. 2019, 60, 1497
3. Britten, T. K.; Kemmitt, P. D.; Halcovitch, N. R.; Coote, S. C. Synlett 2020, 31, 459
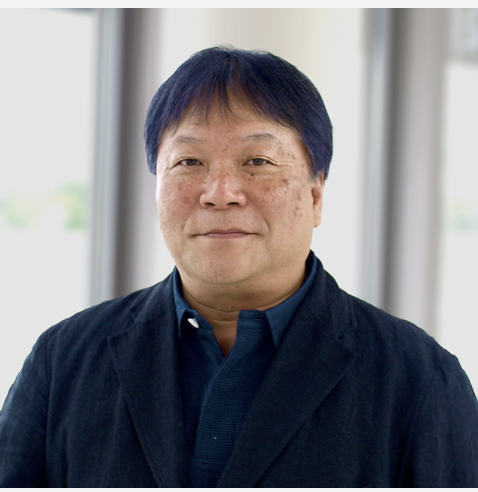
About a century ago, Dr. Hermann Staudinger substantiated the existence of ultralong molecules and won the longterm debate against the colloidal theory to establish polymer science. Needless to say, polymer science has made tremendous contributions to the progress of human society, although it coincidentally brought about a critical environmental issue to tackle.
In his talk, Professor Aida will present the significance and applications of supramolecular polymerization, a modernized version of the colloidal approach to polymeric materials. Supramolecular polymers attract attention not only because they are 100% recyclable but also they can be designed to be environmentally friendly, self-healable, responsive, and/or adaptive [1–4]. In 1988, Prof. Aida and his team reported the first prototype of supramolecular polymerization, featuring the formation of a 1D polymeric assembly using an amphiphilic porphyrin with water-soluble oligoether side chains as the monomer and have made fundamental contributions to this field. Representative examples include (1) nanotubular upramolecular polymerization, (2) chain-growth supramolecular polymerization, (3) supramolecular block copolymerization, (4) stereoselective supramolecular polymerization, and (5) thermally bisignate supramolecular polymerization. These contributions are integral elements of conventional polymer science, filling in the critical gap between supramolecular and conventional polymerizations. Furthermore, Prof. Aida's team have expanded the basic concept of supramolecular polymerization into the noncovalent design of innovative soft materials. Successful examples include the developments of (i) bucky gels, (ii) aquamaterials, (iii) mechanically robust self-healable materials, (iv) supramolecular polymers of biomolecular machines, (v) ferroelectric columnar liquid crystals, and (vi) reorganizable and adaptive core-shell columnar liquid crystals. Prof. Aida will highlight some of these examples to show the significance of supramolecular polymerization for the realization of sustainable society.
REFERENCES
[1] Aida, Meijer, Stupp, Science 2012, 335, 813–817.
[2] Aida, Meijer, Israel J. Chem. 2020, 60, 33–47.
[3] Aida, Adv. Matter. Essay, 2020, 1908140.
[4] Hashim, Bergueiro, Meijer, Aida, Prog. Polym. Sci. 2020, 101250.
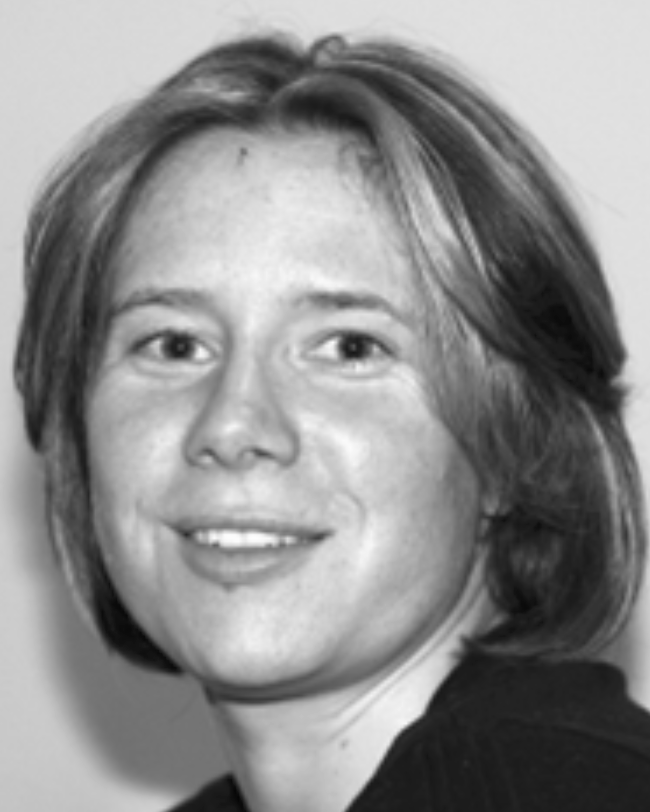
Protic ionic liquids (PILs) are cost efficient “designer” solvents which can be tailored to have properties suitable for a broad range of applications1-2. More recently ionic liquids (ILs) are being combined with molecular solvents, and these binary or multicomponent IL solvents enables more control over the solvent environment, and typically reduces the cost and viscosity.
However, there are relatively few structure-property studies which look at these more complex mixtures. We have explored the solvation properties of common PIL-molecular solvents using various techniques, including Kamlet-Aboud-Taft parameters determined from solvatochromic dyes, and MD simulations3-4. These have identified many interesting solvent properties of these solutions and gives insight into their interactions with solutes. This lead to the use of PIL-water solvents having the potential to control protein solubility and stability, which in turn are critical for applications in bioprocessing, protein crystallography and cryopreservation. In this presentation I will cover some solvation properties of PIL solvents, along with the protein structure changes of proteins in IL solvents which we have explored using spectroscopic and advanced small angle x-ray scattering (SAXS) methods5.
References
1. Greaves, T. L.; Drummond, C. J., Protic ionic liquids: properties and applications. Chem. Rev. 2008, 108 (1), 206-237.
2. Greaves, T. L.; Drummond, C. J., Protic ionic liquids: evolving structure−property relationships and expanding applications. Chem. Rev. 2015, 115, 11379-11448.
3. Yalcin, D.; Christofferson, A. J.; Drummond, C. J.; Greaves, T. L., Solvation properties of protic ionic liquid – molecular solvent mixtures. Phys. Chem. Chem. Phys. 2020, 22, 10995-11011.
4. Yalcin, D.; Drummond, C. J.; Greaves, T. L., Solvation properties of protic ionic liquids and molecular solvents. Phys. Chem. Chem. Phys. 2020, 22, 114-128.
5. Han, Q.; Smith, K. M.; Darmanin, C.; Ryan, T. M.; Drummond, C. J.; Greaves, T. L., Lysozyme conformational changes with ionic liquids: Spectroscopic, small angle x-ray scattering and crystallographic study. Journal of Colloid & Interface Science 2020, DOI: 10.1016/j.jcis.2020.10.024.
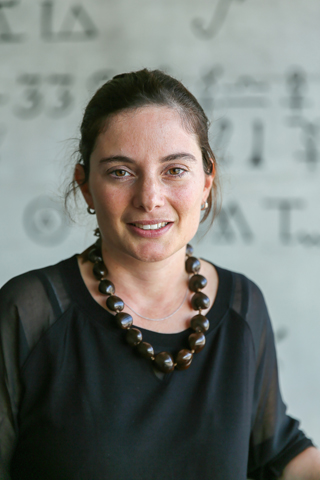
Sabatier’s principle,[1] developed in the first decades of the 20th century, states that an ideal catalyst should bind a substrate neither too strongly nor too weakly. Today, this simple idea provides the fundamental underpinning for “volcano plots”,[2,3] which are abundantly used in heterogeneous and electrocatalysis.[4]
Recently, Prof. Corminboeouf's group have elaborated a computational toolkit[5] based on Sabatier's principle that facilitates the identification of overarching trends present in the “catalysis space” and provides new insights in the best places to “look” for homogeneous catalysts having high activity.
For a given reaction, prospective species anticipated to exhibit enhanced activity can be identified and accompanying design principles that should be useful for synthetic chemists constructed. The combination of these tools with supervised machine-learning techniques enable the expansion of the pool of examined catalysts to over 105 species.
The large quantity of data generated can be compiled and mined into an interactive tool, which facilitates the analysis and assists in identifying the most compatible metal/ligand family combinations with the goal of establishing relationships between the intrinsic chemical properties of different catalysts and their overall catalytic performance. Prof. Corminboeouf's group focus on both prototypical classes reactions and challenging organic processes and highlight some of the pro and cons of the overall approach.
References
[1] P. Sabatier, Ber. Dtsch. Chem. Ges. 1911, 44, 1984.
[2] H. Gerischer, Bull. Soc. Chim. Belg. 1958, 65, 506.
[3] R. Parsons, Trans. Faraday Soc. 1958, 54, 1053.
[3] J. K. Nørskov, T. Bligaard, J. Rossmeisl, C. H. Christensen, Nat. Chem. 2009, 1, 37.
[4] M. D. Wodrich, B. Sawatrlon, M. Busch, , C. Corminboeuf, Acc. Chem. Res. 2021, 6, 6754.
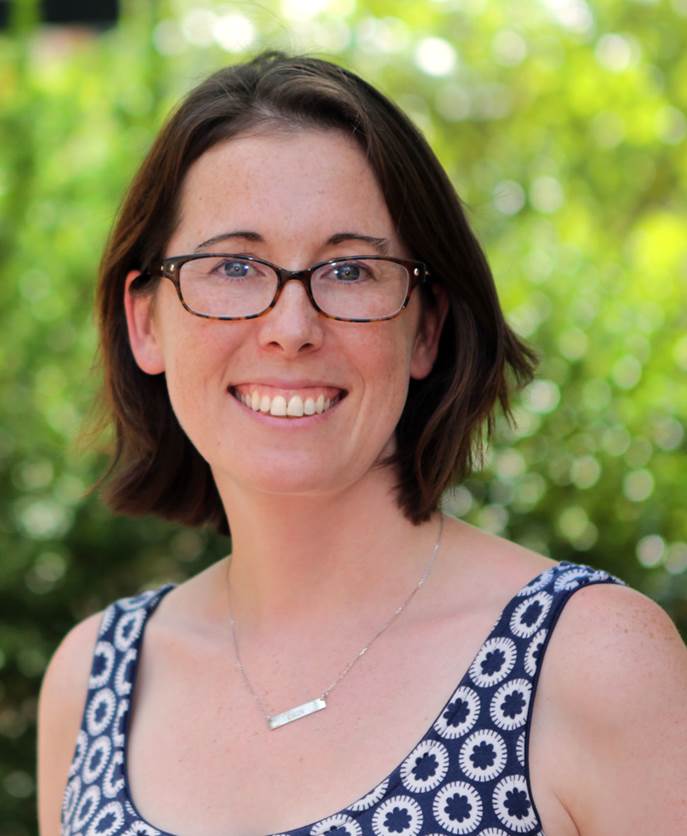
Elucidating Proton-Coupled Electron Transfer Mechanisms Underpinning the Catalytic Generation of Renewable Fuels
The conversion of energy-poor feedstocks like water and carbon dioxide into energy-rich fuels involves multi-electron, multi-proton transformations. In order to develop catalysts that can mediate fuel production with optimum energy efficiency, this complex proton-electron reactivity must be carefully considered.
Using a combination of electrochemical methods and time-resolved spectroscopy, we have revealed new details of how molecular catalysts mediate the reduction of protons to dihydrogen and the experimental parameters that dictate catalyst kinetics and mechanism. Through these studies, we are revealing opportunities to promote, control and modulate the proton-coupled electron transfer reaction pathways of catalysts.
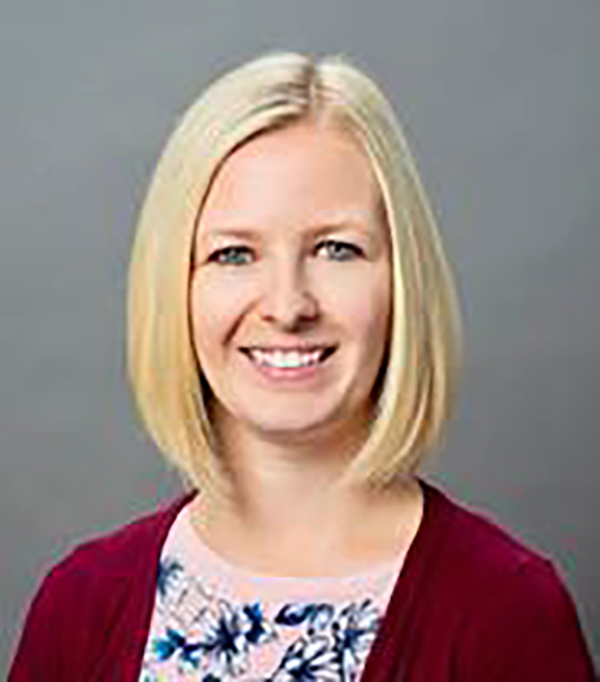
Ionic liquids (ILs) have attracted significant interest as replacement solvents in electrochemical experiments over the last two decades. They possess several properties make them attractive compared to traditional solvent/electrolyte combinations, including high chemical and thermal stability, wide electrochemical windows, low volatility, high polarity, high viscosity and the ability to dissolve a wide range of compounds. My group and I are interested in employing ionic liquids with low-cost planar electrode devices for the detection of target analytes including gases (e.g. oxygen, hydrogen, ammonia, sulfur dioxide, chlorine, methylamine) and explosive compounds (e.g. 2,4,6-trinitrotoluene (TNT), hexahydro-1,3,5-trinitro-1,3,5-triazine (RDX), and octahydro-1,3,5,7-tetranitro- 1,3,5,7-tetrazocine (HMX)).
In this presentation, Dr Silvester-Dean will discuss some of her recent developments in this area, focussing on: (1) the sensing of gases in ILs with different humidity environments, revealing that ion structuring at the electrode is disrupted in the presence of moisture, (2) mixing with polymers to physically stabilise the IL on the electrode, a move towards robust wearable sensors, and (3) the preconcentration ability of ionic liquids that allows the detection of explosive compounds such as TNT using liquid/liquid partitioning. Our long-term goal is to develop a new generation of low-cost commercially available sensors comprising of ionic liquids or gelled electrolytes, for the fast and reliable detection of analytes.
---
Assoc. Prof. Debbie Silvester is an electrochemist and ARC Future Fellow in the School of Molecular and Life Sciences at Curtin University, Perth. She completed her DPhil (PhD) at the University of Oxford, UK, then spent a short time as an intern for Schumberger Cambridge Research, before arriving at Curtin University as a Curtin Research Fellow. In 2012, she was awarded an ARC Discovery Early Career Research Award (DECRA) and in 2017, an ARC Future Fellowship.
Her research focusses on the application of ionic liquids as electrolytes in electrochemical reactions, with an emphasis on understanding fundamental behaviour to develop new electrochemical sensors. She has won various awards for her research, including a Young Tall Poppy award, the Rennie Memorial medal from the Royal Australian Chemical Institute, the Peter W. Alexander Medal from the Analytical & Environmental Division of the RACI, and the Alan M Bond medal from the Electrochemistry Division of the RACI. She is currently the secretary for the Electrochemistry Division of the RACI, and the Australia/New Zealand representative for the International Society of Electrochemistry.
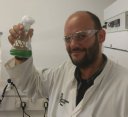
Harmful blue-green algae (cyanobacteria) can pose significant challenges to drinking water treatment with instances of increased biomass and harmful secondary metabolites in the raw water. Conventional water treatment processes can often be challenged and even overwhelmed by harmful algal blooms (HABs).
Advanced oxidation processes in general and semiconductor photocatalysis particularly can represent a solution to the challenges posed by HABs. With an iterative approach to designing and testing a novel UV-LED TiO2-based treatment system we were able improve the raw water quality in a Brazilian reservoir in a pilot scale study.
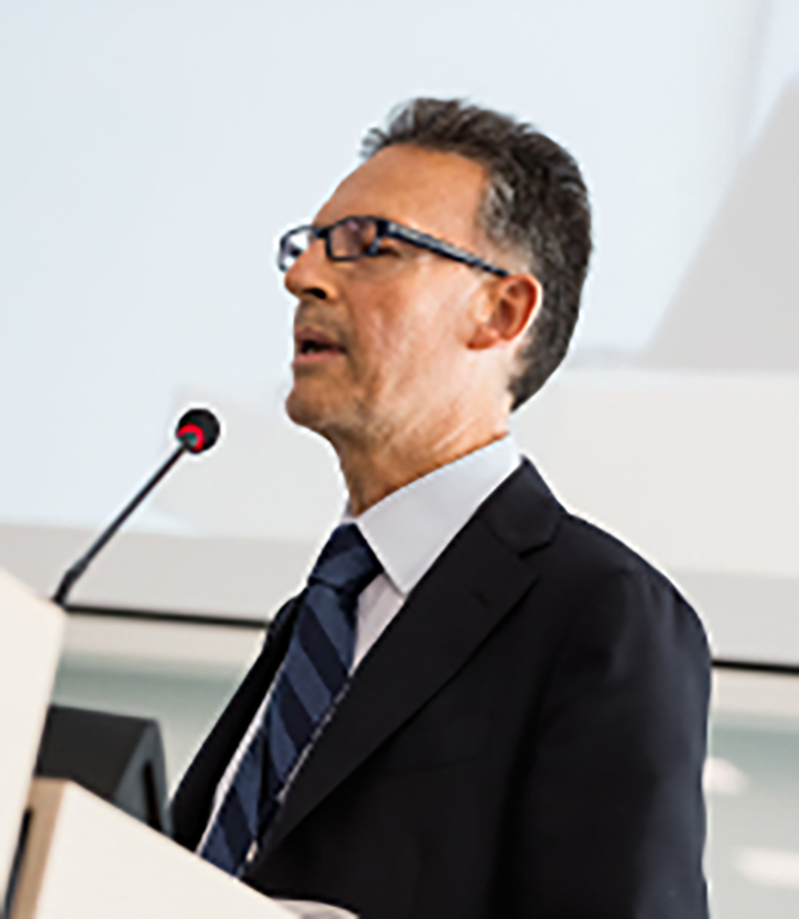
In order to reduce the CO2 emissions and comply with international agreements, a strong increase in the use of renewable energy and in the carbon capture technologies is foreseen. Renewables are however intrinsically fluctuating sources, and this leads to periods of power overproduction which needs to be effectively stored. A valid solution is provided by the Power to Gas (PtG) technology. This process uses the excess power to split water and produce H2 (and valuable O2), which is then reacted with CO2 to form CH4 and H2O through the Sabatier reaction. Ni-based catalysts are used for COx methanation, but from a process intensification standpoint highly active Ru is of interest.
In this presentation the reactivity of Ru/Al2O3 catalysts in the PtG process will be investigated. The effect of the metal loading, of the active phase distribution and of the size of the catalytic support is considered, revealing insights on the optimal Ru loading and on the onset of transport limitations, which is relevant for process intensification where almost complete CO2 conversion is needed in compact reactors. The goal is to develop a once-through process producing a gas stream able to meet, without further purifications other than water removal, the stringent purity thresholds required for the direct injection in the gas grid. The reactivity of Ru-based catalysts in the combined CO2 capture and methanation process is also analyzed.
The idea is to capture CO2 from a CO2-containing stream (e.g. combustion flue gases) with a catalytic material (Dual functioning Material, DFM) that acts as a solid adsorbent, and then exposing the CO2-saturated material to a renewable H2-containing stream in order to produce CH4 and regenerate the adsorbent. In this context, mechanistic aspects involved in the CO2 storage and reduction to methane are analyzed by using a model Ru- Me (Me: K, Na, Ba, Ca, Mg) bifunctional catalysts. The interplay between the adsorbent material and the Ru active phase is investigated, and mechanistic implications are discussed.
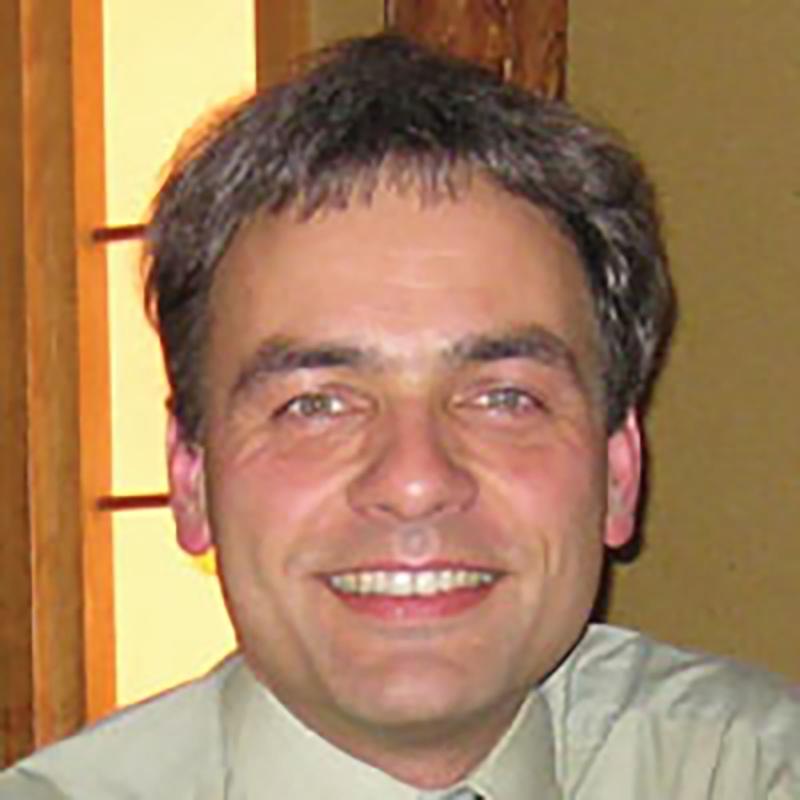
The direct electron transfer at electrode surfaces is one of the prototypical green technologies of the future and the use of microreactors further advances and simplifies that technology.
The development of a robust and flexible flow microreactor for electrochemistry will be discussed alongside applications in synthesis. Combination with subsequent reactions and inline reaction monitoring allow a rapid integration into synthesis. First examples of stereoselective iodine(III)-mediated reactions will be discussed.
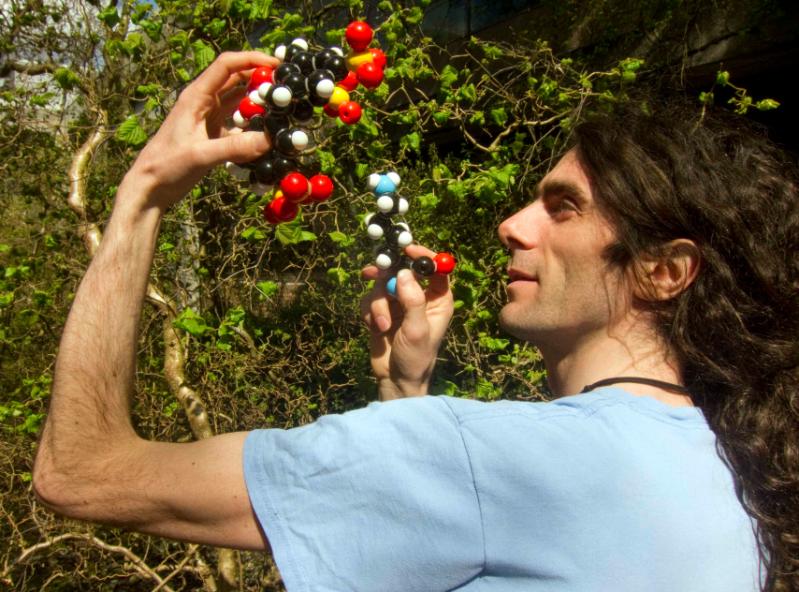
Precisely defined protein aggregates as exemplified by crystals have applications in functional materials. Consequently, engineered protein assembly is a rapidly growing field.
Anionic calix[n]arenes are useful scaffolds that can mould to cationic protein surfaces and induce oligomerisation / assembly. Here, we describe the fabrication of different types of protein frameworks via co-crystallization with sulfonato-calix[8]arene. Calixarene-masked proteins act as nodes within the frameworks, displaying octahedral-type coordination in one case. Such supramolecular protein chemistry may benefit the development of biomaterials and therapeutics.
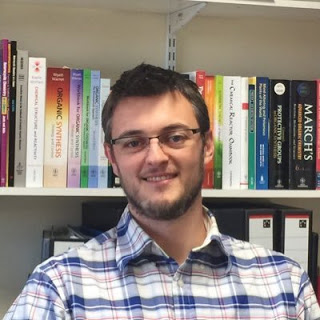
This seminar will focus on the techniques of continuous processing and mechanochemistry/ball-milling in the context of organic chemistry and, through collaboration, their application to metal-free catalysis, synthesis of pharmaceuticals, agrochemicals and macrocyclic fragrances.
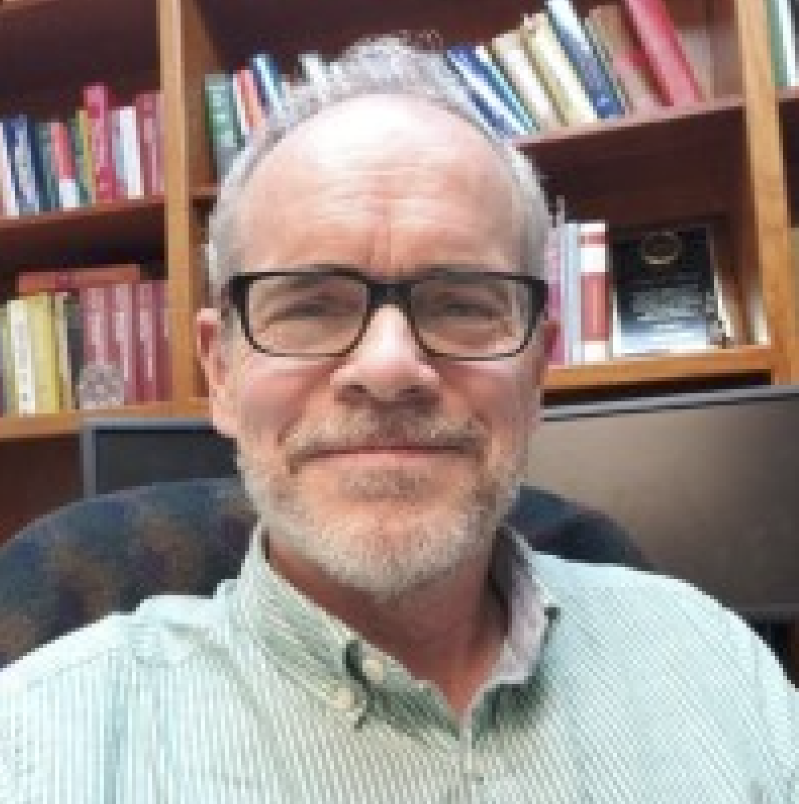
Ammonia (NH3) is a very important molecule with conflicting roles. On the one hand, NH3 is a commodity chemical; its manufacture is integral to the production of fertilizers and polyamides. The oxidation of NH3 on Platinum to NOx (x = 1, 2) is one of the oldest commercialized catalytic reactions; it is the basis for the Ostwald Process for the manufacture of nitric acid. On the other hand, NH3 has emerged as an important reagent in emission control. For the first generation of catalytic converters, NH3 was an inconvenient byproduct and converter design changes were made to minimize its production. For the modern diesel vehicle, NH3 is the NOx reductant of choice. However, due to NH3 toxicity, the NOx reduction must be accomplished without releasing NH3 to the environment by Pt-catalyzed NH3 oxidation. While the Ostwald process relies on high NOx yield, the diesel emission control application requires a high N2 yield.
In this talk we will describe our research in the understanding and design of the structured ammonia slip catalyst (ASC), wherein an NH3 oxidation function is combined with a selective catalytic reduction (SCR) function. We show that a combination of targeted experiments and modeling enable convergence to an optimal architecture that minimizes the Pt loading We will also describe advances in the understanding and modeling of NH3 oxidation kinetics, linking the environmental and chemical manufacturing applications. Finally, we will present a novel core-shell catalyst that comprises a Pt/Al2O3 core and a SCR shell provide for unparalleled ammonia oxidation activity and N2 selectivity.
Dr. Michael P. Harold is the ChBE Chair Professor and Department Chair of Chemical and Biomolecular Engineering at the University of Houston. With expertise in reaction engineering and catalysis, Harold is the author of more than 175 peer-reviewed papers and book chapters and has given over 350 presentations and invited lectures. He is the founder and principal investigator of the University of Houston’s Texas Center for Clean Engines, Emissions & Fuels (TxCEF), established in 2003. Mike was appointed Editor-in-Chief of the AIChE Journal in 2012, the 7th in the Journal’s 60-year history.
A Pittsburgh, Pennsylvania native, Harold received his Bachelor’s degree at Pennsylvania State University, and his PhD from the University of Houston. He joined the faculty at University of Massachusetts at Amherst in 1985 where he became Associate Professor. In 1993 Harold joined DuPont Company, where he held several technical and managerial positions. In 2000 Harold returned to the University of Houston where he became the Dow Chair Professor and Department Chair, a position he held for 8 years. He was re-appointed to that position in early 2013. His honors include the Excellence in Applied Catalysis from the Southwest Catalysis Society in 2019, the Ester Farfel Award at the University of Houston in 2013 (highest honor bestowed on a faculty member) and the American Chemical Society’s Fuel Division Richard A. Glenn Award in 2008.
Professor Harold's research summary can be viewed here: http://www.chee.uh.edu/faculty/harold
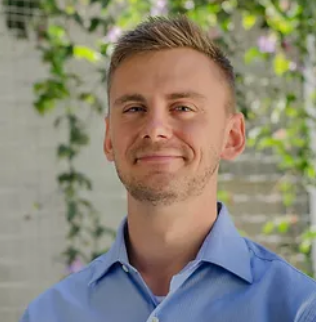
Bioactive natural products (also known as secondary metabolites) have an integral role in biology and ecology as well as modern drug discovery and medicine. The McKinnie lab is interested in understanding how natural products are biosynthesized by identifying and characterizing key enzymes involved in their construction.
One particular enzyme family of interest is the vanadium-dependent haloperoxidases (VHPO); these enzymes catalyze the two-electron oxidation of aqueous halide ions to facilitate regio- and enantiospecific halogenation of organic substrates. Currently characterized actinobacterial VHPO homologs are involved in assembling the chemical scaffolds of a variety of meroterpenoid antibiotics with significant activities against multi-drug resistant bacterial pathogens. This seminar will highlight the chemical history of VHPO enzymes, give insight into the versatile range of chemical reactions catalyzed and variable distribution across biology, and suggest future directions towards their adaptation and evolution for chemoenzymatic syntheses.
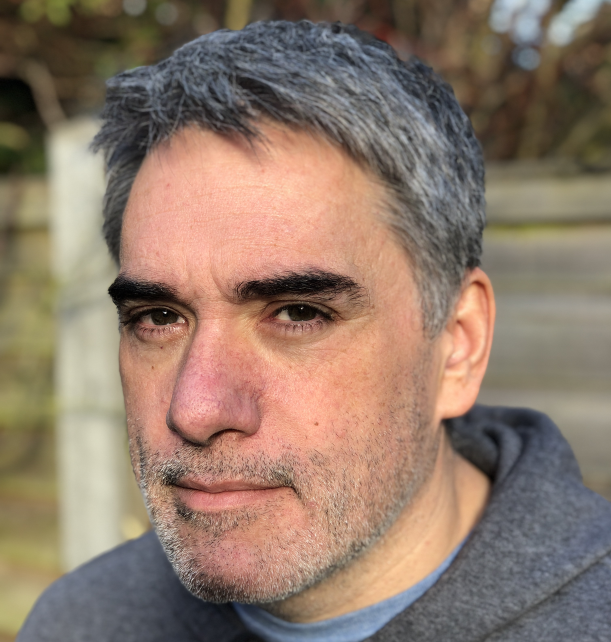
This talk will take a behind-the-scenes look at Nature Chemistry’s editorial processes, as well as providing some guidance on how to write a paper, how to write an abstract and some DOs and DON'Ts when it comes to titles and graphical abstracts – there will also be some advice on how best to appeal an editorial decision. In addition, there will be a broader consideration of peer review in general, the wider chemistry publishing landscape and also other aspects related to scientific publishing including metrics (impact factor, altmetrics, and so on) and the use of social media.
Stuart Cantrill is a graduate of the University of Birmingham in the UK, Stu obtained his PhD in chemistry from UCLA in 2001 (working with Fraser Stoddart), followed by postdoctoral research at Caltech (working with Bob Grubbs). In 2003, he returned to UCLA to tackle a number of different roles, including lecturer, research associate, administrative consultant to the California NanoSystems Institute, and his first job in journal publishing — running an editorial office for the ACS journal Organic Letters. In 2006, Stu returned to the UK to join Nature Publishing Group (now Springer Nature) where he was first an associate and then senior editor at Nature Nanotechnology. In early 2008, he was appointed to be the founding Chief Editor of Nature Chemistry, which launched in April 2009. His main research interests were in the fields of supramolecular chemistry, self-assembly processes and interlocked molecules. But he’s now fascinated by scholarly communication — particularly in chemistry — and how it might be changing in the not-too-distant future. He (very occasionally) blogs about chemistry, gin and related topics at Chemical Connections (http://stuartcantrill.com) and probably tweets a bit too much.
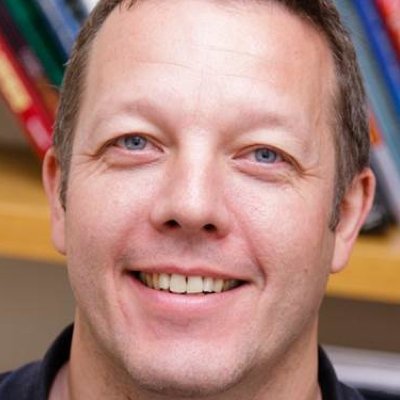
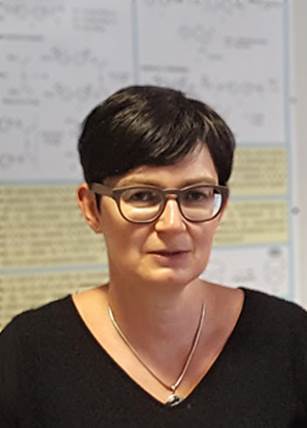
Cyclodextrins (CD) are a well-known family of cyclic oligosaccharides obtained from the enzymatic degradation of starch1 . They possess a truncated conical structure with different cavity sizes. Their relatively hydrophobic cavity associated to the hydrophilic outer surface, enable them to form inclusion complexes in aqueous solution with a wide range of molecules of low hydrophilicity and suitable geometrical size through noncovalent host−guest interactions. Hence, CD can find applications in numerous domains like pharmaceutics, cosmetics, food, catalysis or environmental protection.
We studied extensively inclusion complexes with numerous volatile compounds (VOC, aroma, essential oils) in aqueous solutions and developed various methods to characterize these inclusion complexes2,3. We recently investigated the possibility to combine the properties of CD and deep eutectic solvents (DES)4 . DES are a new generation of green solvents reported for the first time in 20035 . The term DES is commonly used to describe low melting point liquids formed by combining organic salts and hydrogen-bond donor (HBD) components. We were able to solubilize various CD in the DES based on choline chloride:urea (1:2) and demonstrated that inclusion properties of CD were maintained in this DES4,6. To this date, few examples of inclusion complexes formation were reported in solvents other than water. Finally, low melting mixtures were obtained by mixing levulinic acid and various CD derivatives. CD also retained their inclusion ability in the resulting solvent. These new systems gave rise to a new family of solvents that we called SUPRADES7,8. In this seminar, we’ll present an overview of our main results.
References
1 G. Crini, S. Fourmentin, É. Fenyvesi, G. Torri, M. Fourmentin and N. Morin-Crini, Environ. Chem. Lett., 2018, 16, 1361–1375.
2 A. Ciobanu, D. Landy and S. Fourmentin, Food Res. Int., 2013, 53, 110–114.
3 M. Kfoury, L. Auezova, H. Greige-Gerges and S. Fourmentin, Anal. Chim. Acta, 2016, 918, 21–25.
4 T. Moufawad, L. Moura, M. Ferreira, H. Bricout, S. Tilloy, E. Monflier, M. C. Gomes, D. Landy and S. Fourmentin, ACS Sustain. Chem. Eng., 2019, 7, 6345–6351.
5 A. P. Abbott, G. Capper, D. L. Davies, R. K. Rasheed and V. Tambyrajah, Chem. Commun., 2003, 70–71.
6 M. E. Di Pietro, G. Colombo Dugoni, M. Ferro, A. Mannu, F. Castiglione, M. Costa Gomes, S. Fourmentin and A. Mele, ACS Sustain. Chem. Eng., 2019, 7, 17397–17405.
7 T. El Achkar, L. Moura, T. Moufawad, S. Ruellan, S. Panda, S. Longuemart, F. X. Legrand, M. Costa Gomes, D. Landy, H. Greige-Gerges and S. Fourmentin, Int. J. Pharm., 2020, 584, 119443.
8 T. El Achkar, T. Moufawad, S. Ruellan, D. Landy, H. Greige-Gerges and S. Fourmentin, Chem. Commun., 2020, 56, 3385–3388.