Current Projects
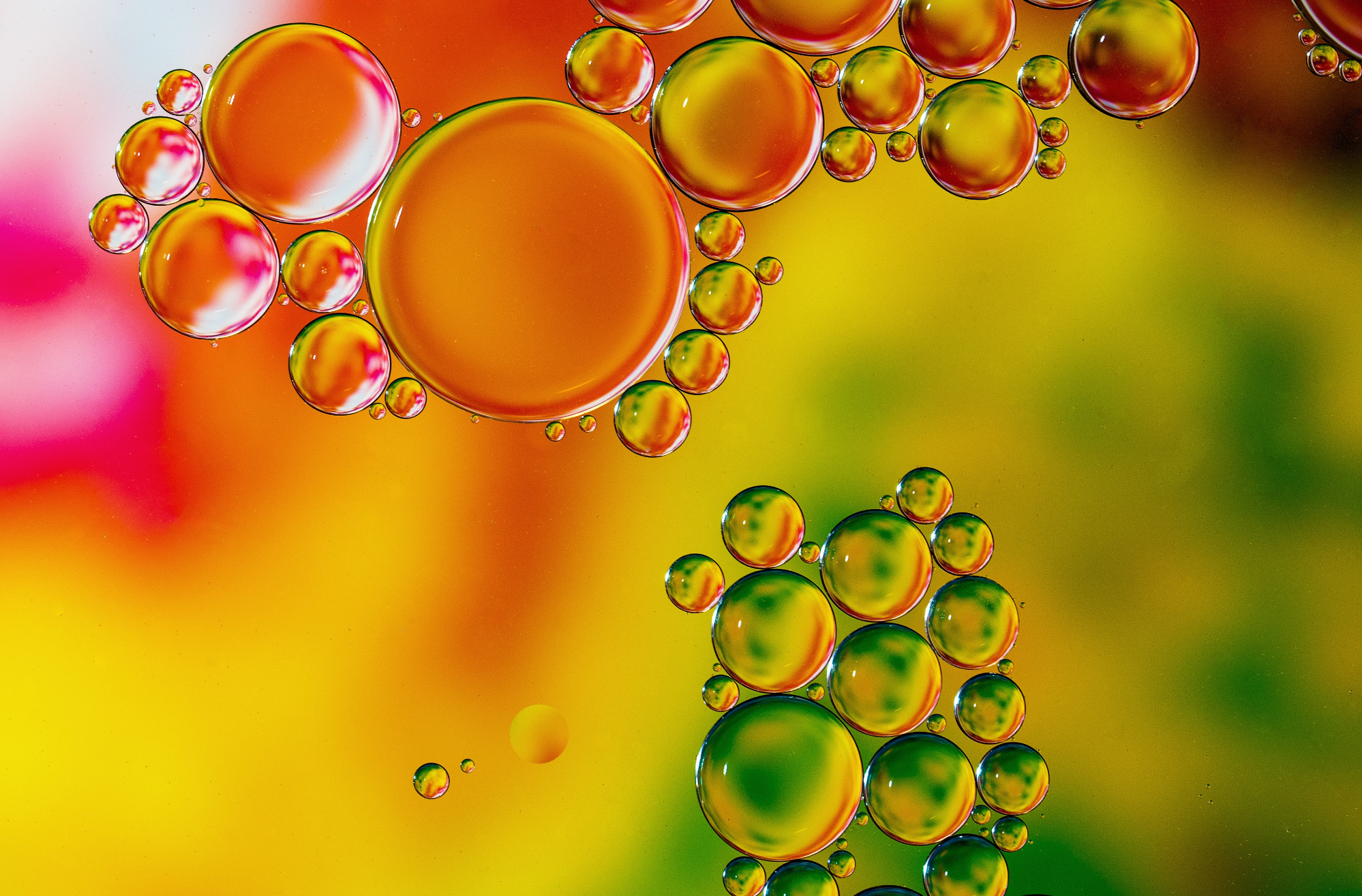
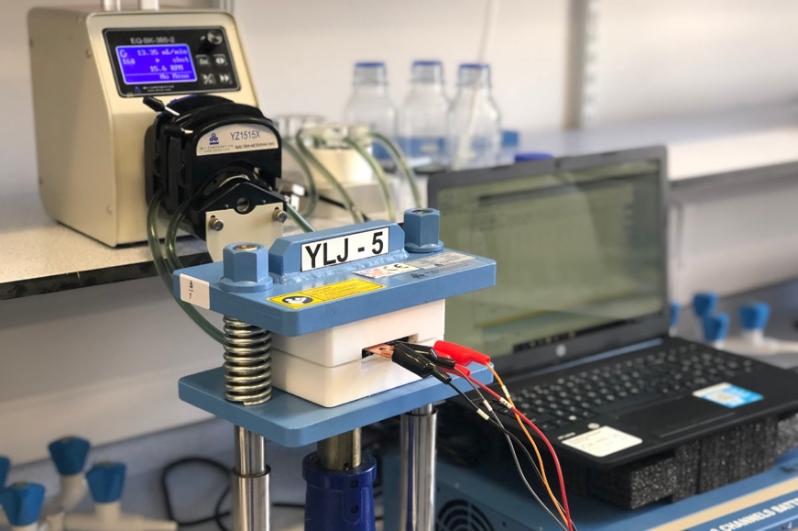
AESIR is a 2-year, CASE-funded project to develop and build a high-TRL “plug & participate”, compact, safe, low-cost, modular redox flow battery prototype of 3.5 kW / 10 kWh for incorporating redox couples employing abundant, non-vanadium chemistries. This will be a collaborative effort between the schools of CCE (Prof Peter Nockemann, PI), MAE (Dr. Stephen Glover and Dr Rob Watson), and EEECS (Dr Tim Littler). Industrial partners of the project are SHELL, Seren AG and Grants Electrical Services.
Renewable energy resources, such as solar, wind, tidal, and biomass, are essential to combat the ongoing challenge of fossil fuel use and global climate change. However, these sources produce intermittent output, limiting their full capacity from being harnessed and used. A prime candidate for storing energy on a grid scale is the Redox Flow Battery (RFB), due to its scalability, room temperature operation, and long cycle life (lifetime >30 years, compared to ~5 years for lithium). An RFB can out-perform and under-cost other battery types, but most RFB technology currently relies on vanadium technologies, an element that is non-abundant in Europe, increasingly expensive, and environmentally harmful to extract.
An energy storage system of this size would be particularly attractive to rural and agricultural users in Northern Ireland, where the national grid is unreliable, facilitating optimisation of micro-renewable infrastructure (e.g. wind, energy, solar, anaerobic digesters). However, RFBs are also an enormously growing market for domestic applications. Due to the modular nature of the proposed system, this can also be easily scaled up to larger energy storage solutions such as wind farm storage or grid peak-shaving – applications which also have huge potential for enabling fossil fuel divestment.
Supported jointly by CASE, Shell, Seren Technology and Grants Electrical Services.
![]() | ![]() |
---|---|
![]() |
![]() |
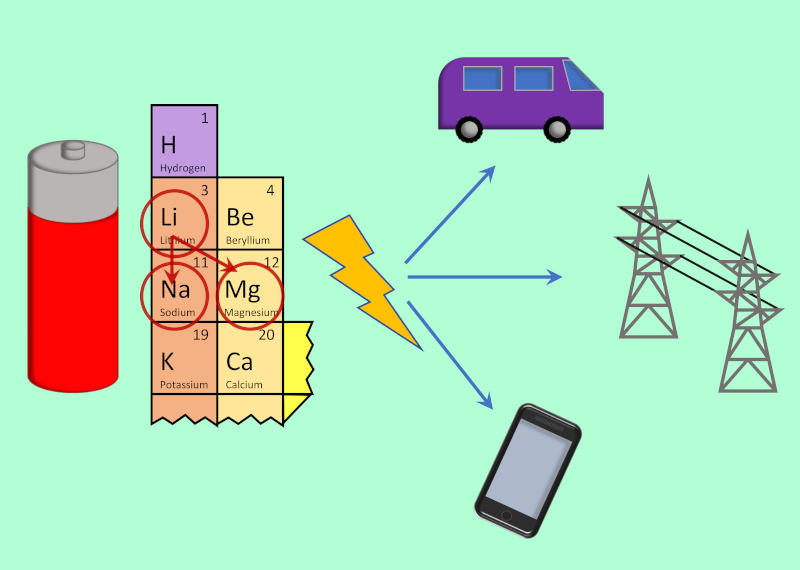
Sustainable energy storage technology for transport applications.
Energy storage solutions are key to bridging the gap between the wealth of renewable power available, and the limitations of when it is available. Past research has often focused on batteries employing liquid electrolytes and lithium charge carriers, but these devices are often faced with issues of safety (leakage, flammability), limited lifetimes and resource availability (e.g. lithium and cobalt).
Research by the combined groups of Prof Nockemann (CCE) and Dr Glover (MAE) and Dr Istrate (MAE) investigate alternative energy materials and electrolytes to provide improved safety and allow for the use of more abundant elements, such as sodium or magnesium, as charge carriers. Bespoke battery cells for in situ and operando investigations provide insight into the uses of these materials in applications such as grid stabilisation and in electric vehicles, and their potential to offer safer, faster-charging and longer-lasting rechargeable batteries.
Supported by Horiba-MIRA Ltd.
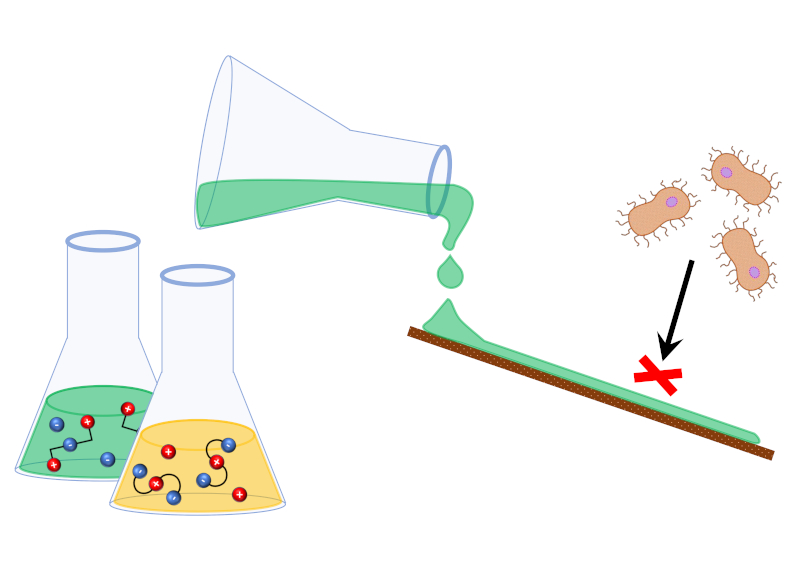
Marijana Blesic and co-workers investigate the potential of zwitterionic polymers to resist attachment of proteins for use in anti-fouling surfaces.
Anti-fouling surfaces are a critical requirement for a wide range of biomedical applications, with unwanted attachment of proteins posing a risk of medical complications with patients or of medical device failure. Although it suffers drawbacks such as susceptibility to oxidation, such surfaces are typically achieved with poly(ethylene glycol) (PEG) films. However recently zwitterionic polymers have shown promise as an alternative, due to their higher chemical stability and comparible biofouling resistance. Zwitterionic salts (ZWSs) are a novel class of compounds that incorporate an additional zwitterionic moiety (a localised positive and negative charge on a single molecule) onto either the anion or cation of a conventional salt. Like ionic liquids they offer many possibilities to tailor properties to a given task or incorporate into polymers and substrates. However ZWSs exhibit pronounced salting in/out ability that lends itself to anti-fouling properties. Understanding the complex equilibria of water/polymer/ZWS and water/protein/ZWS ternary systems would provide crucial information for this industrially important system.
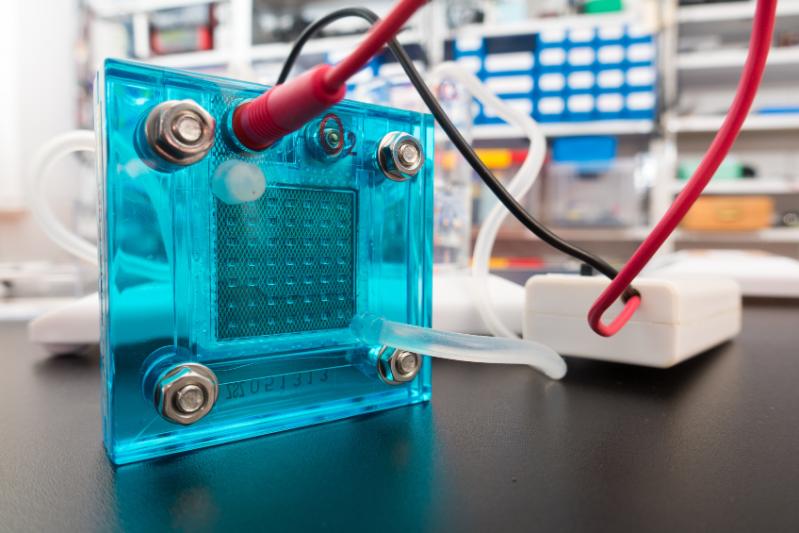
Increased use of renewable energy sources, such as wind or solar power, is vital to prevent climate disaster. However, these sources of energy are intermittent (e.g., sunlight is only available in the daytime) and non-dispatchable (i.e., it cannot be shipped). This necessitates an increase in global energy storage capacity of ca. 1000 GWh by 2030. To address seasonal fluctuations in supply and demand of energy, the world is turning to green hydrogen as a potential solution. The most efficient way to convert hydrogen back to electricity is via a fuel cell, which generates voltage from the separate reactions of hydrogen and oxygen, giving water as the only by-product. The most common fuel cell type is the low-temperature polymer electrolyte fuel cell (PEFC) that uses platinum electrocatalysts and hydrated perfluorinated membranes. However, their commercial adoption is limited by the high cost of these materials and the low tolerance of today’s PEFCs to fuel impurities.
A nascent alternative technology is the intermediate-temperature PEFC that runs at elevated temperatures with respect to the incumbent technology. As a result, IT-PEFCs have a greater tolerance to fuel impurities, benefit from improved kinetics, and can be run with other hydrogen carriers, such as methanol. This technology can be unlocked by the implementation of a range of ionic liquids, which have low volatility but high inherent ionic conductivity.
This project is led by Josh Bailey, a new Vice-Chancellor Illuminate Fellow, and aims to combine the chemical synthesis of poly- and protic ionic liquids with the assembly and engineering of fuel cells to achieve high-efficiency energy conversion whilst reducing water management issues found in traditional devices. This includes the development of new ionomer materials used in catalyst layers and the production of ionogel membranes with the ideal properties to advance this exciting new technology.
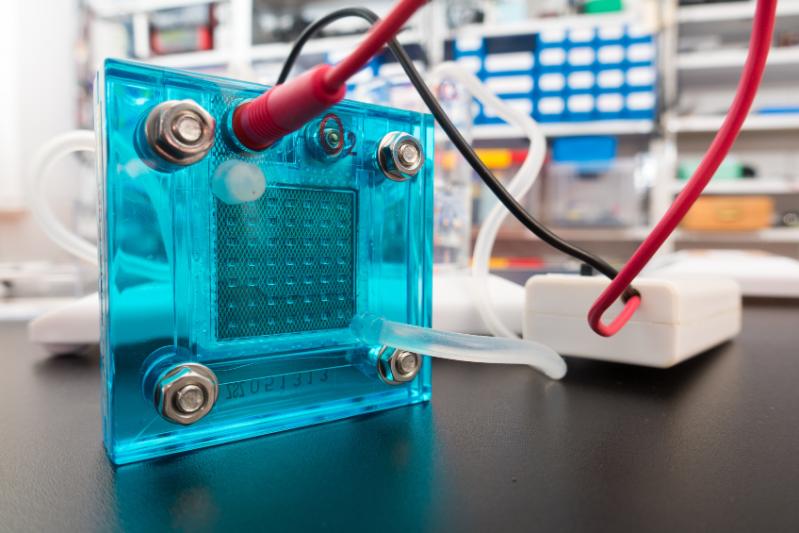
Our lab is invested in developing next-generation electrochemical devices for clean energy applications. Our research centers on three key areas: fuel cells for power generation, electrolysers for hydrogen production from water, and electrochemical hydrogen pumps for efficient compression.
To achieve breakthroughs in these technologies, we're particularly interested in utilising ionic liquids as novel electrolytes. These designer salts hold promise due to their exceptional stability, inherent conductivity, and the ability to tailor their properties. By incorporating ionic liquids as electrolytes, we aim to create fuel cells, electrolysers, and hydrogen pumps that boast improved efficiency, durability, and performance.
Supported by CASE:
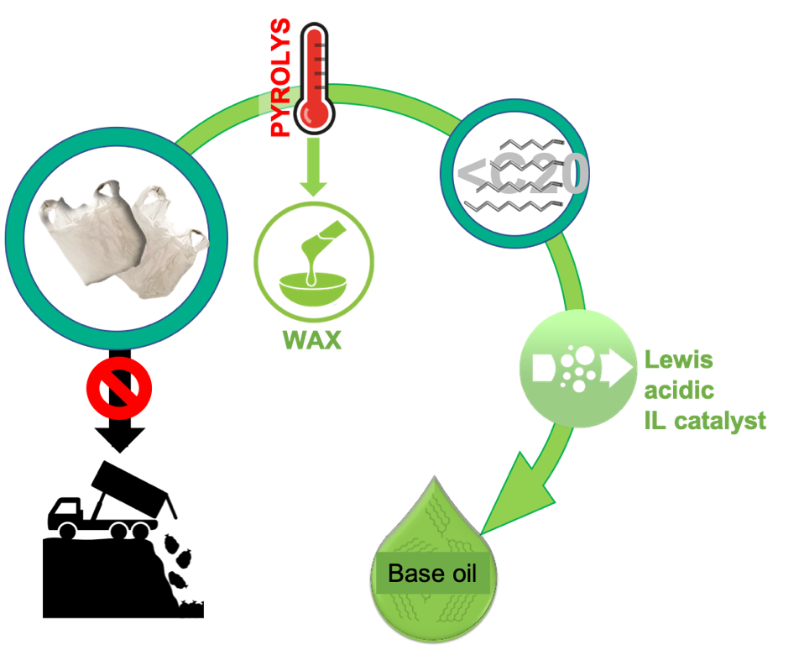
Plastic waste that contaminates the environment, in particular the oceans, is now considered one of the key challenges by the EU funding bodies and the UK government alike. In June 2018, the UK Science Minister announced a £20 million Plastics Research and Innovation Fund to stimulate the development of new technologies for plastic removal and recycling, as well as development of more sustainable plastics. There is a strong drive to abandon the current model of “make, use and dispose”, moving to a new circular economy model: “reduce, reuse and recycle”. But - what can be done on a practical level to achieve this change?
An increasingly popular technology is pyrolysis of plastics to fuels (the first plant was commissioned in 2000 in Japan, now there are about 90 across the world). In pyrolysis, waste polyolefin plastics: plastic bags, containers and packaging – are subjected to high temperatures (>400°C), which causes their decomposition to light hydrocarbon fuels: syngas, gasoline, kerosene and diesel, in addition to carbon char. Although this technology produces fuel from waste, it has two crucial drawbacks:
- Economic: low added value of fuels, considering the cost of plastic collection, sorting and pyrolysis
- Environmental: burning fuels generates CO2 to atmosphere, therefore shifting the environmental burden into a different area, rather than eliminating it
A more recent approach, is plastic pyrolysis to waxes instead of fuels; most of waste plastic polyolefins get converted to heavy hydrocarbons (more than 20 carbons in the chain) called waxes, used as waterproofing agents, packaging, candles, personal care products, viscosity modifiers, mound release agents etc. They are high added-value products, and because they are not burned for fuels – do not contribute to CO2 emissions.
In this Invest Northern Ireland-funded project, Dr Swadźba-Kwaśny and her group are looking to valorise the major by-product in plastics-to-was process: the <C20 olefin-paraffin fraction. Using Lewis acidic ionic liquids, they aim to convert the mixed light olefins to base oils: the main component for synthetic lubricants. Ultimately, all olefins generated from waste plastics will be transformed into high added-value products, and none of it will be burned as CO2-emitting fuel.
Supported by Invest Northern Ireland:
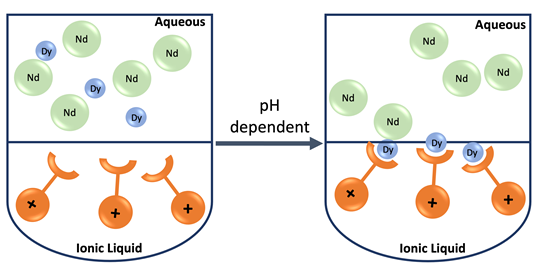
Prof Peter Nockemann at QUILL, together with his research group led by Dr Ritesh Ruhela developed in 2014 a breakthrough innovative ionic liquid-based extractant for the separation of REEs. Combining their expertise in rare-earth separation, coordination chemistry, and ionic liquids, they designed a novel functionalized ionic liquid-based extractant, MAIL (Multifunctional amide ionic liquid), for the selective liquid-liquid extraction of REEs.
Ionic liquids not only have great potential as alternative and more benign solvents but, in addition, the ability to fine-tune their structures enables specific functionality to be imparted. As a result, a more environmentally friendly extractant with superior selectivity was designed. Initial extraction focused on the separation of neodymium/dysprosium from ‘end-of-life’ hard disc drives containing NdFeB magnets.
Coupling research to innovation, the first patent on this work was filed in 2016 (granted in 2020). In the same year, Seren Technologies was founded, led by managing partners Edward Morrell and Tom Bucknall and the exclusive license to develop and commercialise the ionic liquid technology for rare-earth separation was secured. The prime determinant of any move toward industrial scale is understanding the economy of scale. After initial proof-of-concept and trials of separation technologies e.g. mixer-settlers and centrifugal extractors at Queen’s University Belfast, an independent techno-economic evaluation and conceptual design was implemented to ensure economic viability.
In 2018, Seren Technologies opened its first pilot plant in The Wilton Centre, Teesside. Here, their team of chemists and a chemical engineers optimised the synthesis scale-up of the ionic liquid extractant, developed methods for the pre-treatment of ‘end-of-life’ magnetic scrap, and further explored the process conditions for the extraction technology. During this time an additional four process patents were filed. Three PhD chemistry students and two master’s students were sponsored by Seren to continue innovation and research at Queen’s University Belfast. This has enabled the development of second generation extractants, and the computational modelling of the separation process.
With support from Seren Technology:
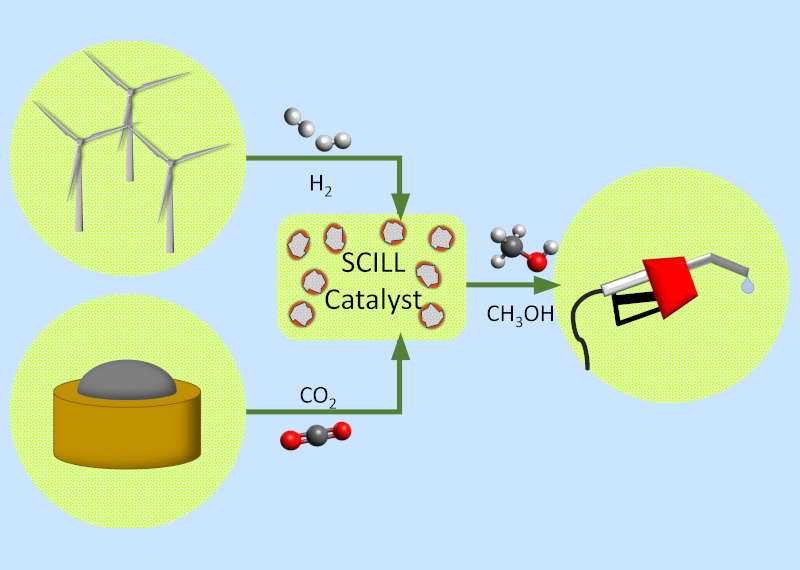
Development of a SCILL catalyst to utilise gas from AD reactors for the production of green methanol.
With its new applications in alternative fuels (e.g. fuel cells, DME and LPG), there is a huge potential market for methanol production. This project is investigating the possibility of using biogas from anaerobic digestor (AD) plants (640 in the UK) in conjunction with hydrogen produced from a curtailed wind farm (270 in the UK) to produce methanol from a completely renewable source. This green source of methanol has the scope to provide an addition £2 million to the UK economy through production of non-petrochemical fuel.
The project is collaboratively headed by Nancy Artioli and Gosia Swadźba-Kwaśny, and aims to combine both chemical and engineering strategies to improve current gas-to-methanol production methods. This will include development of a new solid catalyst with an ionic liquid layer (SCILL) in conjunction with a novel micro-channel fixed bed reactor.
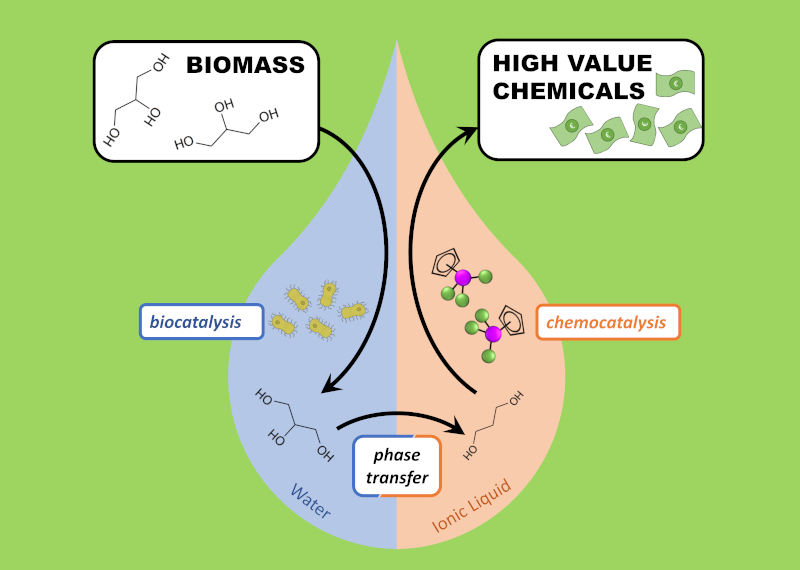
Andrew C. Marr and Patricia C. Marr are investigating applications of ionic liquids in biocatalysis and renewables valorisation.
Ionic liquids have many unique properties that can be applied to invent innovative technological solutions to industrial problems.
Ionic liquids have been shown to stabilise proteins. The addition of ionic liquids to enzymes, and synthetic biocatalysts such as artificial metalloenzymes, can improve the separation and recycling. Bio-derived catalysts can be entrapped within an ionic liquid gel in order to further improve their ease of use.
The tuneable solvent properties of ionic liquids can also be exploited. Ionic liquids can be prepared that are not miscible with water, yet have a high ability to dissolve and extract polar solutes. Coupled with their biocompatibility, this allows bioprocesses to be operated with a layer of ionic liquid that extracts the product as it is formed.
The low volatility of ionic liquids can also assist in bioprocess and renewable separations. In reactions that increase the volatility of the substrate chemical, operating in an ionic liquid enables the reaction to be run under vacuum, thus separating the product from the catalyst as it is formed.
Supported by EPSRC Catalysis Hub and the EU FP7 project GRAIL: