Archive
It is a well-known fact that if two liquids are mixed in a container their polarity difference will determine the intermolecular forces between the molecules and it will ultimately lead to two possible outcomes: formation of a miscible or partially miscible mixture. Qualitative and quantitative information on the miscibility of two or more compounds in a system is a starting and key requirement for at least one stage in almost every process in chemistry and chemical engineering including the design of reaction media, efficient extraction systems, or formulations in pharmaceutical, food, and cosmetic industries.
While in a huge number of cases experimental determination of miscibility can be done very simply by a visual method, this project will be focused on the experimental investigation of miscibility in the systems in which the visual method fails to provide accurate information on the number of phases present in the system or when an inconsistency between results obtained using different methods was manifested. The following techniques will be employed to complement the understanding of underlying phenomena in the studied mixtures: refractive index, fluid phase equilibria, and light-scattering measurements, and simulation using COSMOTherm software.
The project outcome should put some light on the limitations of different techniques and call for caution in their applications.
Anticipated outcome:
Potential outcome could be a manuscript submitted to Journal of Chemical Education (ACS).
Project Duration:
12 weeks.
For more detail and to apply, please contact directly Dr Marijana Blessic (m.blesic@qub.ac.uk).
As the demand for and implementation of renewable energy grows, so does demand for solutions which can store this energy to regulate when it is used.
A major cost factor and limitation for the next generation of redox-flow batteries based on vanadium is the electrolyte, which is often the issue for the lower energy densities of VRBs in comparison to other battery types. By using new non-aqueous, ionic liquid-based formulations containing redox-stable ionic liquids containing a dispersion of nanoparticles, we can currently increase the vanadium concentration in the electrolyte.1 An overall improvement of the vanadium concentration will lead to a significantly improved energy density that is currently one of the limitations of these redox-flow battery systems. In this project, we will further develop the electrolyte chemistries and characterise them. The electrolytes will then be electrochemically characterised and tested and evaluated in a redox flow cell.
Figure 1: Schematic view of a vanadium redox flow battery (left). Redox flow cell (right).
You will have the opportunity to work as a part of a research team and acquire a very broad set of skills: advanced inorganic synthetic methods, combined with analytical techniques such as UV/Vis, IR/Raman, NMR, PXRD, single-crystal XRD as well as electron microscopy (SEM) for material characterisation. A student who likes coordination chemistry, nanomaterials and has an interest in (moderate) (in)organic synthesis would be ideal.
Reference: L. Bahadori, R. Boyd, A. Warrington, M.S. Shafeeyan, P. Nockemann, Evaluation of ionic liquids as electrolytes for vanadium redox flow batteries, J. Mol. Liq., 2020, 317, 114017.
Anticipated outcome:
This work follows up on a prevous project and aims to gather more data for finishing up a publication and is expected to result in an EPSRC grant application.
Duration (up to 12 weeks) and orientational dates:
8 weeks
For more detail and to apply, please contact directly Prof Peter Nockemann (p.nockemann@qub.ac.uk)
Mixer-settlers are devices to contact and separate two immiscible liquids and have been a part of the metal extraction and recovery process for decades. As extraction and purification of different metals in metal extraction plants becomes more essential, so does the need to look into new ways of manufacturing the mixer settlers.
3D printing is an emerging technique which offers the user the freedom of form and design and which can be easily used in a range of applications. The most common 3D printing techniques are fused deposition modeling (FDM) and stereolitography (SLA). Additive manufacturing can have applications on a number of chemical process unit operations including pumps, mixers, and reactors (P.A. Kozak, 2020). Many of the factors which determine the total performance of the mixer-settler with respect to size and separation are the same as those which affect the mass transfer performance of the mixer (A.D. Ryon, 1960). The mass transfer performance and phase separation operations are significantly affected by independent variables such as: mixer configuration, agitator speed, residence time in mixer, phase continuity, organic/aqueous ratio, specific settler flow, and settler configuration.
Figure 1: (a) CAD model of 3D printable mixer settler and (b) 3D printed mixer settler unit.
References: P. A. Kozak, P. Tkac, K. E. Wardle, M. A. Brown, G. F. Vandegrift, 2020. Demonstration of the MOEX Process Using Additive-Manufacturing-Fabricated Annular Centrifugal Contactors, Solvent Extraction and Ion Exchange; A.D. Ryon, F.L. Dley, R.S. Lowries, 1960. Design and scale-up of mixer-settlers for the dapex solvent extraction process.
Anticipated outcome:
This work follows up on a prevous project and aims to gather more data for finishing up a publication and is expected to lay the ground for a grant application.
Duration (up to 12 weeks) and orientational dates:
8 weeks
For more detail and to apply, please contact directly Dr Oana Istrate (O.Istrate@qub.ac.uk)
Catalytic oxidation is a class of commercially important reactions. Organic sulfoxides and sulfones formed via the oxidation of sulfides are important compounds in the pharmaceutical and flavour industry. Conventionally these oxidations have been carried out in volatile organic solvents, however the use of ionic liquids (ILs) have received great deal of attention as possible “green” replacement mainly due to their non-measurable vapor pressure. Additionally, hydrogen peroxide has been increasingly used as an environmentally benign oxidant for several catalytic reactions.
This project will focus on the use of novel ionic liquids with metal anions (Cu, Fe, V) which will be used in conjunction with hydrogen peroxide as new catalytic systems for the formation of sulfoxides and sulfones.
Anticipated outcome:
The successful candidate will take part of the publication of a scientific paper.
Duration (up to 12 weeks) and orientational dates:
10 weeks with starting date on 4th July and ending in 9th of September
For more detail and to apply, please contact directly Dr Peter Goodrich (P.Goodrich@qub.ac.uk)
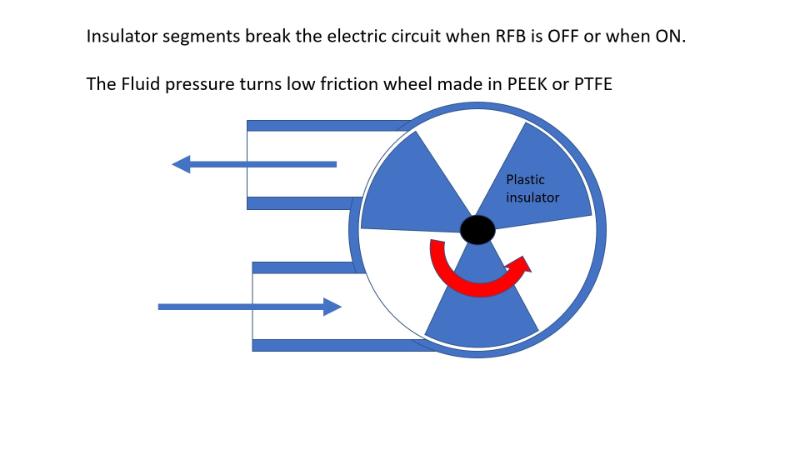
Redox Flow Batteries (RFB) all suffer from a form of internal current or energy leakage known as shunt currents. It’s similar to putting a battery in water and having a short circuit path via the water but to a much lesser extent. To counter the problem current RFB’s have long thin manifolding to create high leakage resistance paths. This unfortunately causes massive pumping losses during RFB use.
To counter the issue in a more effective way the team at QUB wish to investigate a blocker wheel as shown below. At all times the solid parts of the wheel form a physical barrier that disconnects the flow and therefore the energy leakage path. The wheel rotates with the electrolyte flow during RFB use. The wheel does not generate pressure but will take energy to rotate it. The better the wheel is sealed the less energy leakage but the more pump energy is needed to turn the wheel. In that respect the top and bottom of the wheel will be solid and run on a O ring or labyrinth seal (not shown). It’s is hoped that a tight running tolerance will minimise the required operation torque and the thin film of electrolyte on the wheel surface will minimise the energy loss. The aim of the project is to design a series of wheel and test them using saline solution as a conductive electrolyte. The baseline case is no wheel. The project will look to 3 D print the wheel design in an experimental test housing. The wheel needs to minimise shunt current when the RFB is working (with flow) and when shut off.
This work will run alongside the multidisciplinary QUB team’s RFB research in four concurrent PhD’s and one CASE project. No known publications reference any work in this area, so highly novel. High potential IP for QUB and follow-up joint interdisciplinary EPSRC application.
Project Duration: 8 weeks.
For more detail and to apply, please contact directly Dr Stephen Glover (s.glover@qub.ac.uk).
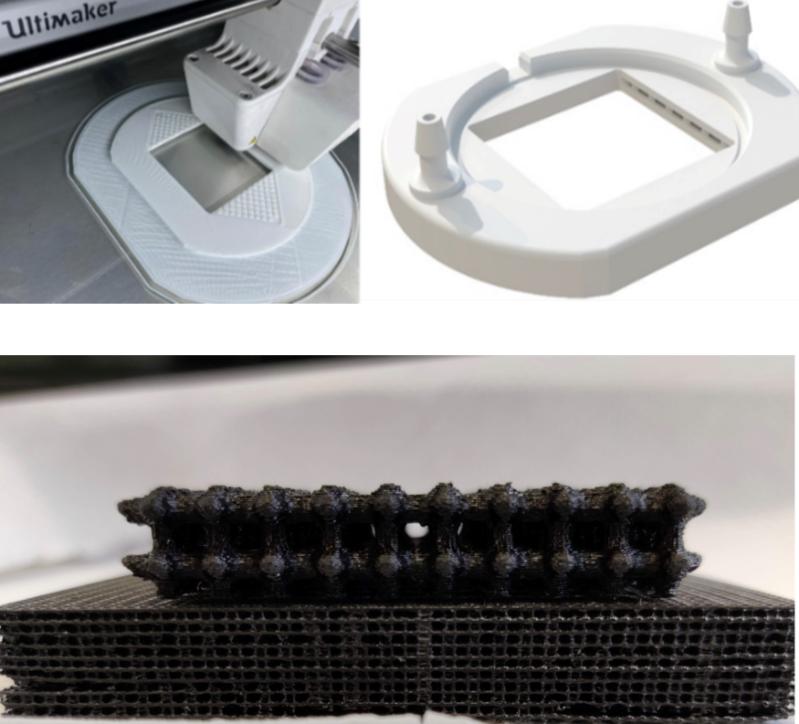
It is critical to the growth of RFBs as a viable technology that development focuses on low-cost and more sustainable electrolytes based on cheaper, earth-abundant redox materials. One of the most important key components of a redox flow battery (RFB) is the electrode. The role of the electrodes is to ensure efficient electron charge transfer and to facilitate the reactions of the electrolyte redox couple on their surface.
This PhD project aims to investigate and improve the electrode/electrolyte interface. The application of post-treatments of the carbon felts, using oxidative activation combined with surface-decoration with nano-electrocatalysts, is expected to yield significant improvements in the electrochemical reversibility and to lower activation overpotential, resulting in higher battery efficiency. The project aims to focus particularly on iron and vanadium-based electrolytes consisting of metal complexes with ligand systems that have so far not been investigated in terms of their electrode / electrolyte interactions. These chemically activated electrodes will be investigated using various in-situ, ex-situ and operando spectroscopic techniques (Raman, FTIR, XPS, EPR) to provide insight into the underlying activation mechanisms.
Work programme and objectives
The effects of degree of compression and treatment of the electrode materials will be investigated to improve the electrode/electrolyte interface and increase the power density of the redox flow batteries. This studentship will aim to establish the relationship between degree of compression, porosity, and electrolyte transport, as well as investigating the relationship between electrode treatment, permeability, and electrolyte transport. Different compression rates and treatments (e.g., chemical treatments and/or thermal treatments) will be investigated using a combination of electrochemical impedance spectrometry (EIS) and load curve measurements applied at different states of charge. The electrode / electrolyte interface of treated electrodes and carbon felts will be investigated under operando conditions by using complementary in-situ and ex-situ spectroscopic techniques (specifically Raman spectroscopy) and transmission electron microscopy (TEM), scanning electron microscopy (SEM) and micro-computed tomography (CT) techniques in bespoke 3D-printed cells.
The PhD student will work in close collaboration with the industrial partner (Shell) and will have the possibility to spend up to 6 months at the Shell Technology Centre Amsterdam (STCA), Netherlands (funded). The student will work there closely with a team of international redox flow battery experts and will have access to Shell’s high-end, state-of-the-art energy and lab facilities. The PhD student will present and discuss progress in monthly meetings with the academic supervisory team and a team of energy storage experts from Shell. These meetings will provide additional technical feedback and an industrial perspective to the research.
The ideal candidate should enjoy working in a multi-disciplinary field of energy storage that ranges from materials to chemistry and phyiscs. Team-working qualities, clear communication skills and the ability to learn and develop new techniques are key for a successful candidate. Co-supervisors for this project are Prof Peter Nockemann (CCE) and Dr Miryam Arredondo-Arechavala (MAP).
Funding Information
UK studentships - cover tuition fees and include a maintenance stipend of £17,668 per annum, together representing an investment in your education of more than £65,000.
A UK studentship is open to UK and ROI nationals, and to EU nationals with settled status in the UK, subject to meeting specific nationality and residency criteria.
DfE studentship eligibility information can be viewed at: https://www.economy-ni.gov.uk/publications/student-finance-postgraduate-studentships-terms-and-conditions
EPSRC studentship eligibility information can be viewed at: https://www.ukri.org/what-we-offer/developing-people-and-skills/esrc/funding-for-postgraduate-training-and-development/eligibility-for-studentship-funding/
International studentships, where available, will also cover tuition fees and include an equivalent maintenance stipend.
For more information please contact: Dr Oana Istrate (O.Istrate@qub.ac.uk)
Closing date for application: Monday, February 27, 2023
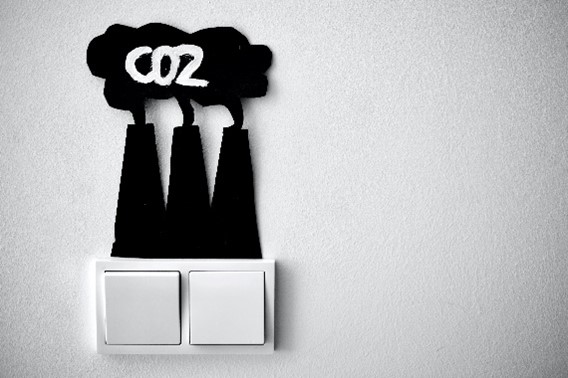
The large-scale conversion of carbon dioxide into synthetic fuels, such as methanol, is an attractive prospect which could alleviate our reliance on fossil fuels and help achieve our net zero goals. To achieve this, carbon dioxide must be converted to a more reactive ‘radical’ form so it that it can be combined with protons and electrons to form energy rich molecules. Naturally, this is achieved by photosynthesis whereby plants, for example, use photoactive enzymes to activate and transform carbon dioxide into carbohydrates, which are then used to fuel cellular processes.
This project aims to develop novel synthetic routes from ‘carbon dioxide to fuels’ utilising simple electro/photo-active molecular catalysts, such as quinones, which can rapidly transfer protons and electrons to carbon dioxide, mimicking the catalytic activity of photo-enzymes.
Training in all electrochemical, photochemical, and structural characterisation techniques necessary to complete the project will be provided. Candidates should have, or expect to achieve, a first or upper second-class honours degree or equivalent in chemistry or chemical engineering. It is recommended that interested students should contact Dr Paul Kavanagh to discuss the position in more detail before making a formal application.
For more information please contact: Dr Paul Kavanagh (P.Kavanagh@qub.ac.uk)
Closing date for application: Friday, February 24, 2023
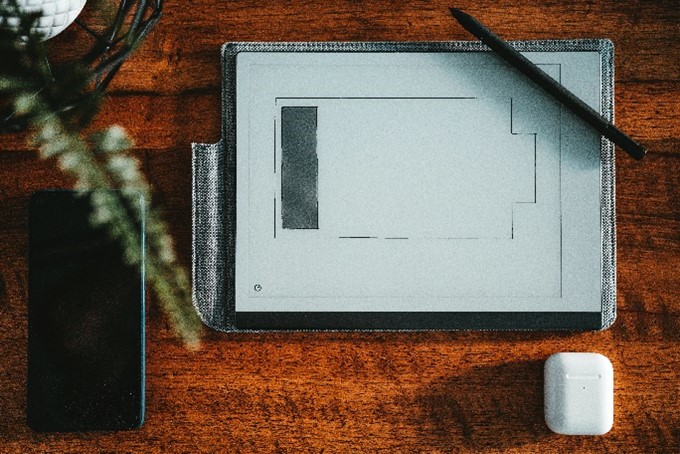
Redox flow batteries (RFB) are a promising technology for stationary energy storage systems which will become a real necessity as we transition to a low-carbon energy landscape that relies more heavily on intermittent renewable energy. In such RFBs, the electrical energy is stored in liquids through redox reactions of dissolved redox couples at electrodes.
The choice of electrolytes is a key factor in the costs, durability and sustainability of these batteries, and currently prohibits the wider implementation of this promising technology. Suitable, sustainable electrolytes must have a good balance between stability and solubility of the ions in the oxidation states involved, enable rapid and reversible electrode reactions and must consitst of compounds with high availability and affordability of raw materials. Chelation of metal complexes has demonstrated the ability to modulate redox potential and increase redox kinetics and solubility to generate high performance, low cost RFBs.
This PhD project will focus on the design and synthesis of chelating ligands, their complexation with earth abundant metals as redox couples as electrolytes for RFBs. Based on a proof-of-concept, and with the help of computational modelling (Density Functional Theory, DFT), a range of iron, cerium, manganese and vanadium redox couples with suitable potential difference and reversibility in charge/discharge experiments will be synthesised. According to the criteria for the electrolyte, these need to form stable solutions of abundant metals (Fe, Mn, Ce), using non-flammable, non-toxic solvents and complexing agents which result in high solubility and redox potentials in a suitable range.
By using new redox-couples containing redox-stable metal complexes consisting of earth-abundant metals such as iron, manganese or cerium, electrolytes with performances similar to vanadium but at lower costs can be synthesised. The intention is to explore new electrolyte chemistries and redox couples with non-vanadium chemistries. Thereby, a range of organic ligands will be designed assisted by computational methods (DFT calculations), and the redox potentials of the metal complexes will be determined, resulting in a ‘map’ of complex structures vs. redox potentials. In particular, iron, cerium and manganese and vanadium have redox potentials within the right margin which can form long-term stable complexes; however, the structure / property relationship regarding their electrochemical potential is currently not fully understood. Introducing ligands can alter the redox potentials, and therefore the cell voltage of the RFB. The new electrolyte solutions will be characterised using electrochemical characterisation methods, ranging from CVs and EIS to the characterisation of the ion mobility in the electrolyte.
The PhD student will work in close collaboration with the industrial partner (Shell) and will have the possibility to spend up to 6 months at the Shell Technology Centre Amsterdam (STCA), Netherlands (funded). The student will work there closely with a team of international redox flow battery experts and will have access to Shell’s high-end, state-of-the-art energy and lab facilities. The PhD student will present and discuss progress in monthly meetings with the academic supervisory team and a team of energy storage experts from Shell. These meetings will provide additional technical feedback and an industrial perspective to the research.
The ideal candidate should enjoy working in a multi-disciplinary field of energy storage that ranges from inorganic chemistry, materials chemistry to analytical techniques. Team-working qualities, clear communication skills and the ability to learn and develop new techniques are key for a successful candidate.
For more information please contact: Professor Peter Nockemann (p.nockemann@qub.ac.uk) or Dr Oana Istrate (O.Istrate@qub.ac.uk)
Closing date for applicatio: Friday, February 24, 2023
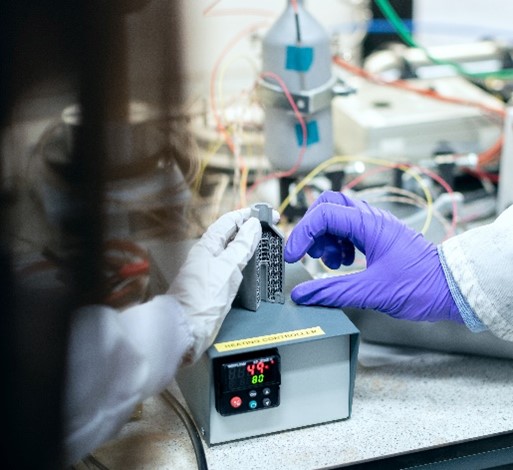
Redox flow batteries (RFB) are a promising technology for stationary energy storage systems which will become a real necessity as we transition to a low-carbon energy landscape that relies more heavily on intermittent renewable energy.
We have recently developed a 3D-printing platform to produce laboratory-scale RFB test cells, demonstrating leak tightness, chemical stability, and versatility with regards to cavity thickness and internal manifold design. Importantly, these cells have demonstrated, through rapid prototyping, improved performance versus a commercially available test cell. Common designs are flow-by and flow-through configurations, the latter of which is the industry standard and the focus of this study.
This PhD project aims to investigate redox electrolyte flow in bespoke miniaturised operando flow cells as a function of internal manifold, compression, flow rate and current density. Customised, high-fidelity 3D-printed cells have been shown to provide excellent leak tightness and chemical compatibility, and their polymeric structure and versatile design make them amenable to high throughput X-ray computed tomography experiments, as well as complementary operando spectroscopic techniques such as XPS, UV/Vis and Raman microscopy. This allows the impact of manifold design and compression on electrolyte utilisation in operating cells to be probed, a remaining challenge in the field towards increasing performance and lowering costs.
The effects of internal manifold design, particularly as a function of cell compression, on the flow distribution and electrode porosity saturation remains poorly understood in an operating cell. This studentship will aim to establish the relationship between macro-parameters, the microporous flow regime, and the electrochemical performance by imaging miniature operando cells with high temporal resolution, appropriate spatial resolution to capture an RVE and with sufficient resolution and contrast to characterise electrolyte flow. In parallel, spectroscopic techniques (UV-VIS, Raman microscopy, EPR) will be conducted. EXAFS at synchrotron facilities (Diamond Light Source, Oxfordshire) will be employed for monitoring the local oxidation state of the redox species. The results of these spectroscopic techniques, together with the computational modelling will then feed into improved cell designs, which will then be experimentally validated.
The PhD student will work in close collaboration with the industrial partner and will have the possibility to spend up to 6 months at the Shell Technology Centre Amsterdam (STCA), Netherlands (funded). The student will work there closely with a team of international redox flow battery experts and will have access to Shell’s high-end, state-of-the-art energy and lab facilities.
The PhD student will receive extensive training and access to this facility over the full time of the studentship, which is a unique opportunity, since access to high-spec micro-CT instruments is normally limited to a few places worldwide, and access & operation are very costly. The student will moreover present and discuss progress in monthly meetings with the academic supervisory team and a team of energy storage experts from Shell. These meetings will provide additional technical feedback and an industrial perspective to the research.
The ideal candidate should enjoy working in a multi-disciplinary field of energy storage that ranges from inorganic chemistry, materials chemistry to analytical techniques, additive manufacturing and aspects of design & engineering. Team-working qualities, clear communication skills and the ability to learn and develop new techniques are key for a successful candidate. Co-supervisors for this project are Dr Oana Istrate (MAE) and Dr Stephen Glover (MAE).
For more information please contact: Professor Peter Nockemann (p.nockemann@qub.ac.uk)
Closing date for application: Friday, February 24, 2023
Deep eutectic solvents have been proposed as low environmental impact solvents to address many challenges, including metal separations and extractions where traditional molecular solvents (especially volatile organic solvent, VOCs) have been perceived as undesirable. New deep eutectic solvents containing trioctylphosphine oxide (TOPO) as a basic hydrogen-bond acceptor have been recently developed within the Queen’s University Ionic Liquids Laboratory (QUILL). These fluids have many desirable properties including a large liquid composition, low viscosity, and good hydrophobicity which allows them to be used for extraction applications from water as alternatives to conventional organic solvents.
This project builds on continued research leading to the development of a hydrophobic DES for the selective extraction of gallium from waste streams. Gallium is an element in critical supply, especially given the recent technological advancements and the provision of 5G networks on a nationwide scale. In order to meet the global demands, more waste sources must be valorised to ensure that a steady supply of gallium can be maintained.
The student will work in the QUILL laboratories and gain experience designing and developing green solvents, as well as using them in extraction processes. A broad range of analytical techniques will be used, in particular thermal measurements: TGA and DSC, in addition to quantifying metal content by XRF and studying metal speciation by vibrational spectroscopy (IR and Raman).
Duration (up to 12 weeks) and orientational dates: 10 weeks
For more detail and to apply, please contact directly Prof Gosia Swadzba-Kwasny (m.swadzba-kwasny@qub.ac.uk)
This project is in continuation of the summer studentship, previously supported by QUILL. So far, we have successfully synthesised the polymer immobilised ionic liquid (PIIL) catalysts and evaluated the activity in cross-Aldol condensation of heptanal and benzaldehyde for production of Jasminaldehyde. We have prepared tetraethyl ammonium piperidine-2-carboxylate as an exemplar amino acid IL and immobilised on Ambersep 900 hydroxide ion exchange resin.
For achieving high yield of jasminaldehyde, it is important to enhance the kinetics of cross-Aldol condensation, and minimise the self-Aldol condensation of heptanal. Both these objectives can be achieved by combining catalyst design and process design. We have prepared active PIIL catalyst and preliminarily evaluated the semi-batch process using heptanal as the limiting reagent. Selectivity of Jasminaldehyde could be significantly improved to 92% in semi batch process, as compared to 70% in batch process.
Over summer 2023, we will further optimise the jasminaldehyde process using semi-batch conditions, and also transform the process to continuous flow conditions using new reactor design, a distributed plug-flow reactor, which is now fabricated and ready to use. As compared to normal plug flow conditions, the distributed plug flow reactor mimicks the semi-batch process, but in continuous flow. Successful completion of the project will allow us to widen the scope of reactions to similar cross Aldol condensations useful in perfumery and flavours such as Raspberry ketone, using environment friendly PIIL catalysts in continuous flow.
Duration (up to 12 weeks) and orientational dates: 12 weeks
For more detail and to apply, please contact directly Dr Haresh Manyar (h.manyar@qub.ac.uk)
This project aims to screen and identify materials based on liquid eutectic mixtures with and without supramolecular enhancers such as cyclodextrins as gas capture and separation media for biogas upgrading.
The goal is to prepare performant materials for CO2 uptake versus CH4. The sorbents will be prepared by a PhD student, Mark Young, who will also supervise and train the student in the relevant techniques. The summer student will perform material characterisation (NMR, viscosity, density, TGA, DSC, Karl-Fischer) and the screening for CO2, CH4 and for CO2/CH4 mixed absorption capacities and separation selectivity via headspace gas chromatography (HS-GC). The results will be compiled and analysed to determine any tendencies and important functionalities for sorbent optimisation.
Duration (up to 12 weeks) and orientational dates: 8 weeks
For more detail and to apply, please contact directly Dr Leila Moura (L.Moura@qub.ac.uk)
Mixer-settlers are devices to contact and separate two immiscible liquids and have been a part of the metal extraction and recovery process for decades. As extraction and purification of different metals in metal extraction plants becomes more essential, so does the need to look into new ways of manufacturing the mixer settlers (figure 1).
3D printing is an emerging technique which offers the user the freedom of form and design and which can be easily used in a range of applications. The most common 3D printing techniques are fused deposition modeling (FDM) and stereolitography (SLA). Additive manufacturing can have applications on a number of chemical process unit operations including pumps, mixers, and reactors (P.A. Kozak, 2020). Many of the factors which determine the total performance of the mixer-settler with respect to size and separation are the same as those which affect the mass transfer performance of the mixer (A.D. Ryon, 1960). The mass transfer performance and phase separation operations are significantly affected by independent variables such as: mixer configuration, agitator speed, residence time in mixer, phase continuity, organic/aqueous ratio, specific settler flow, and settler configuration.
Figure 1: (a) CAD model of 3D printable mixer settler and (b) 3D printed mixer settler unit.
References: P. A. Kozak, P. Tkac, K. E. Wardle, M. A. Brown, G. F. Vandegrift, 2020. Demonstration of the MOEX Process Using Additive-Manufacturing-Fabricated Annular Centrifugal Contactors, Solvent Extraction and Ion Exchange; A.D. Ryon, F.L. Dley, R.S. Lowries, 1960. Design and scale-up of mixer-settlers for the dapex solvent extraction process.
Duration (up to 12 weeks) and orientational dates: 12 weeks
For more detail and to apply, please contact directly Dr Oana Istrate (O.Istrate@qub.ac.uk)
As the demand for and implementation of renewable energy grows, so does demand for solutions which can store this energy to regulate when it is used. A major cost factor and limitation for the next generation of redox-flow batteries based on vanadium is the electrolyte, which is often the issue for the lower energy densities of VRBs in comparison to other battery types.
By using new non-aqueous, ionic liquid-based formulations containing redox-stable ionic liquids containing a dispersion of nanoparticles, we can currently increase the vanadium concentration in the electrolyte.1 An overall improvement of the vanadium concentration will lead to a significantly improved energy density that is currently one of the limitations of these redox-flow battery systems. In this project, we will further develop the electrolyte chemistries and characterise them. The electrolytes will then be electrochemically characterised and tested and evaluated in a redox flow cell.
Figure 1: Schematic view of a vanadium redox flow battery (left). Redox flow cell (right).
The student will have the opportunity to work as a part of a research team and acquire a very broad set of skills: advanced inorganic synthetic methods, combined with analytical techniques such as UV/Vis, IR/Raman, NMR, PXRD, single-crystal XRD as well as electron microscopy (SEM) for material characterisation. A student who likes coordination chemistry, nanomaterials and has an interest in (moderate) (in)organic synthesis would be ideal.
Reference: L. Bahadori, R. Boyd, A. Warrington, M.S. Shafeeyan, P. Nockemann, Evaluation of ionic liquids as electrolytes for vanadium redox flow batteries, J. Mol. Liq., 2020, 317, 114017.
Duration (up to 12 weeks) and orientational dates: 12 weeks
For more detail and to apply, please contact directly Prof Peter Nockemann (p.nockemann@qub.ac.uk)
There are currently no funded postgraduate research vacancies available.
Please check this page regularly for updated information, and be sure to read the information in relation to other opportunities below.
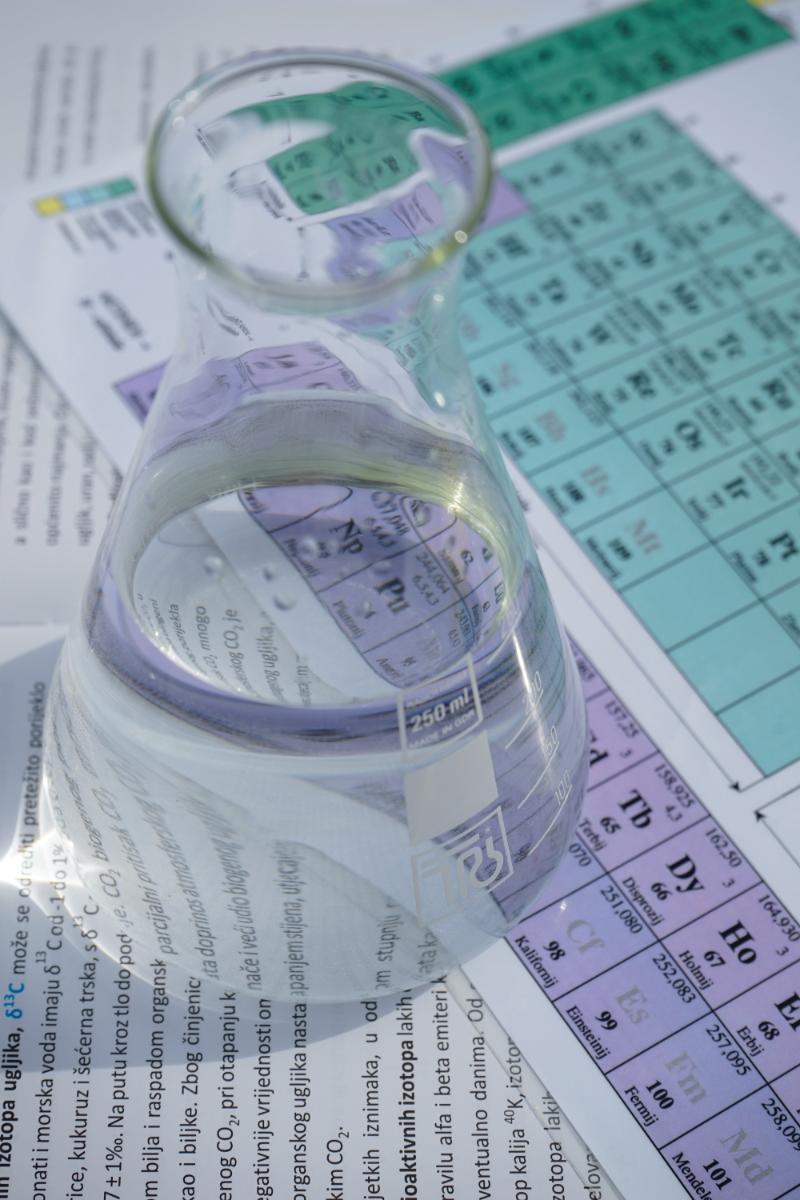
Redox flow batteries (RFB) are a promising technology for stationary energy storage systems which will become a real necessity as we transition to a low-carbon energy landscape that relies more heavily on intermittent renewable energy. In such RFBs, the electrical energy is stored in liquids through redox reactions of dissolved redox couples at electrodes. The choice of electrolytes is a key factor in the costs, durability and sustainability of these batteries, and currently prohibits the wider implementation of this promising technology. Suitable, sustainable electrolytes must have a good balance between stability and solubility of the ions in the oxidation states involved, enable rapid and reversible electrode reactions and must consist of compounds with high availability and affordability of raw materials. Chelation of metal complexes has demonstrated the ability to modulate redox potential and increase redox kinetics and solubility to generate high performance, low cost RFBs.
This PhD project will focus on the design and synthesis of chelating ligands, their complexation with earth abundant metals as redox couples as electrolytes for RFBs. Based on a proof-of-concept, and with the help of computational modelling (Density Functional Theory, DFT), a range of iron, cerium, manganese and vanadium redox couples with suitable potential difference and reversibility in charge/discharge experiments will be synthesised. According to the criteria for the electrolyte, these need to form stable solutions of abundant metals (Fe, Mn, Ce), using non-flammable, non-toxic solvents and complexing agents which result in high solubility and redox potentials in a suitable range.
By using new redox-couples containing redox-stable metal complexes consisting of earth-abundant metals such as iron, manganese or cerium, electrolytes with performances similar to vanadium but at lower costs can be synthesised. The intention is to explore new electrolyte chemistries and redox couples with non-vanadium chemistries. Thereby, a range of organic ligands will be designed assisted by computational methods (DFT calculations), and the redox potentials of the metal complexes will be determined, resulting in a ‘map’ of complex structures vs. redox potentials. In particular, iron, cerium and manganese and vanadium have redox potentials within the right margin which can form long-term stable complexes; however, the structure / property relationship regarding their electrochemical potential is currently not fully understood. Introducing ligands can alter the redox potentials, and therefore the cell voltage of the RFB. The new electrolyte solutions will be characterised using electrochemical characterisation methods, ranging from CVs and EIS to the characterisation of the ion mobility in the electrolyte.
The PhD student will work in close collaboration with the industrial partner (Shell) and will have the possibility to spend up to 6 months at the Shell Technology Centre Amsterdam (STCA), Netherlands (funded). The student will work there closely with a team of international redox flow battery experts and will have access to Shell’s high-end, state-of-the-art energy and lab facilities. The PhD student will present and discuss progress in monthly meetings with the academic supervisory team and a team of energy storage experts from Shell. These meetings will provide additional technical feedback and an industrial perspective to the research.
The ideal candidate should enjoy working in a multi-disciplinary field of energy storage that ranges from inorganic chemistry, materials chemistry to analytical techniques. Team-working qualities, clear communication skills and the ability to learn and develop new techniques are key for a successful candidate. This project will be co-supervised by Dr Oana Istrate (MAE) and Dr Paul Kavanagh (CCE).
Funding Information
This studentship is for immediate start. The studentship is jointly funded by industry and DfE and is open to UK and ROI nationals (with residency conditions), or EU nationals that have obtained settled status in the UK. Full eligibility and stipend information can be viewed via: https://www.nidirect.gov.uk/articles/department-economy-postgraduate-studentship-scheme
For more information please contact: Prof. Peter Nockemann (p.nockemann@qub.ac.uk)
Project length: 3 Years full time
Funding body: DfE CAST Award
Closing date for application: CLOSED
More details and to Apply click Here.
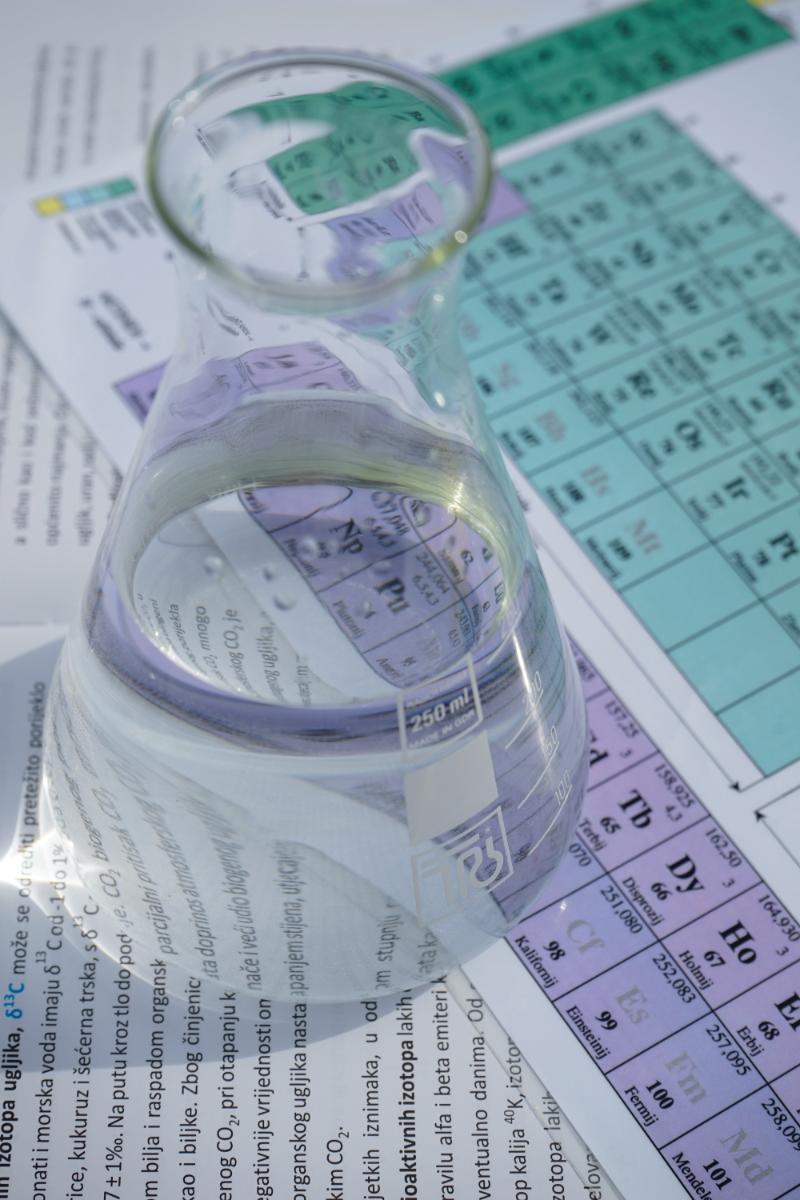
Efficient hydrometallurgical separation of rare earths from NdFeB magnets is critical for their recycling and relies on robust process chemistry. Ionic liquids as extracting additives are a promising solution, promoting better phase separation and increased separation factors, but the mechanism of increasing separation factor is not fully understood, and the cationic structures have not been optimised.
This PhD project aims to develop strategically designed ionic liquid additives that enhance separation and phase partitioning in an existing pilot/demonstration-scale NdFeB recycling process.
Their influence on extraction efficiency, kinetics, interfacial properties and corrosion will be studied. Multi-technique speciation studies (UV-Vis and Raman spectroscopies in house, as well as EXAFS at Diamond Light Source or European Synchrotron Radiation Facility - ESRF) will be used to understand and fine-tune the coordination chemistry, leading to optimised ionic liquid design. The ultimate goal is to deliver high-performance ionic liquid additives that boost separation factors, phase separation, and process robustness. The optimised chemistries will be tested in pilot-scale NdFeB recycling. We expect new insights into the ionic liquid design and rare earth speciation for selective separation from end-of-life magnets.
A PhD candidate with an interest in inorganic and physical chemistry with a strong Chemistry or Chemical Engineering background would be ideal for this project.
Funding Information
** Funding for this project studentship is not guaranteed. Applicants for this and a number of other projects will be in competition for studentships funded by the Northern Ireland Department for the Economy (DfE)**
Full eligibility (including residency conditions) and funding information can be viewed via https://www.nidirect.gov.uk/articles/department-economy-studentships
Candidates must possess or expect to obtain, a 2:1 or first-class degree in Chemistry or closely related discipline
Candidates must be available to start the post by October 2024
For more information please contact: Prof. Peter Nockemann (p.nockemann@qub.ac.uk)
Project length: 3 Years full time
Funding body: DfE (competitive against over projects)
Closing date for application: CLOSED
More details and to Apply click Here.
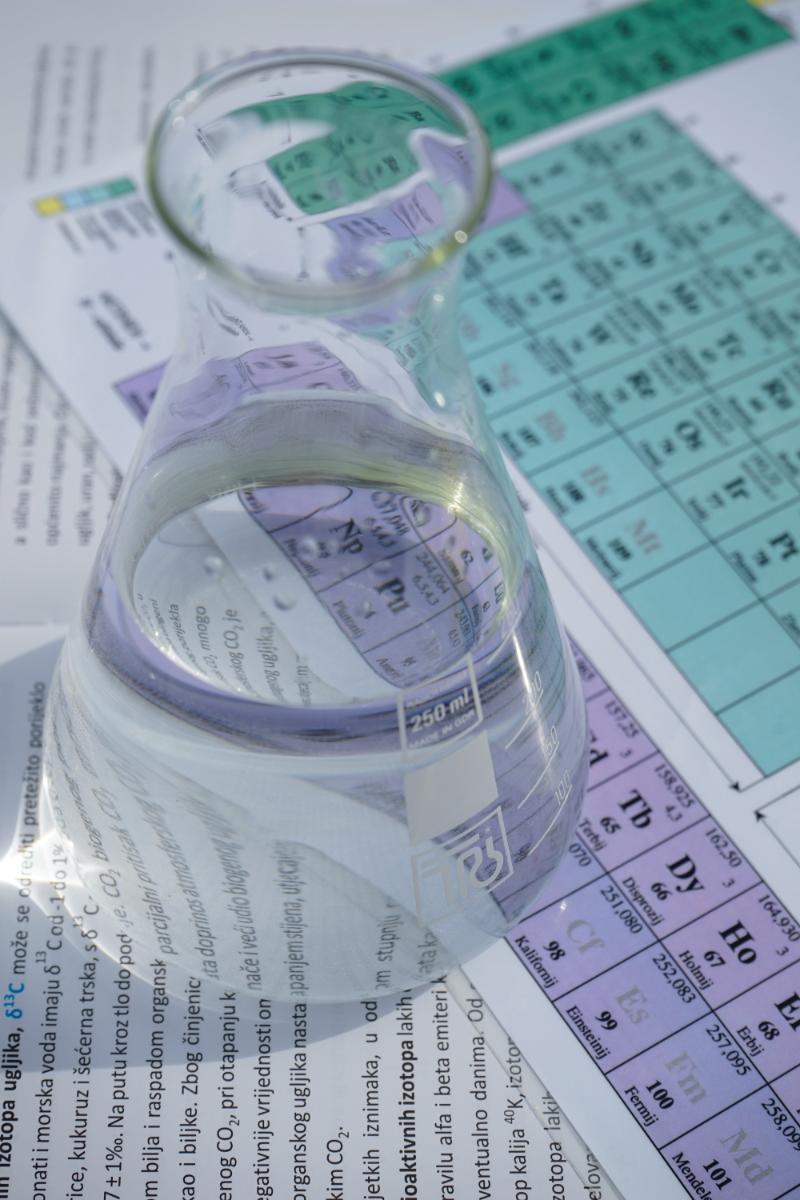
Rare earth elements like samarium and transition metals like cobalt are critical materials used in high-performance permanent magnets found in technologies like headphones, electric vehicles, and wind turbines. Recycling these metals from end-of-life magnets is important for supply sustainability and reducing environmental impacts. However, the separation of samarium from cobalt is a key challenge in recycling; traditionally done using complex, energy-intensive processes. There is a need to develop more efficient and sustainable hydrometallurgical approaches to enable effective recycling of these high-value metals.
This PhD project will comprehensively investigate the development and optimisation of hydrometallurgical processes for the recycling of samarium and cobalt from end-of-life magnets. It will involve an in-depth literature review to identify knowledge gaps in existing methods. Bench-scale experiments will be used to test and refine a hydrometallurgical separation process using real-world magnet waste as feedstock. Advanced techniques like operando EXAFS spectroscopy to understand metal speciation changes under process conditions, in collaboration with Diamond Light Source. Pilot plant trials will demonstrate feasibility for scaled-up implementation.
A PhD candidate with an interest in inorganic and physical chemistry with a strong Chemistry or Chemical Engineering background would be ideal for this project.
Funding Information
** Funding for this project studentship is not guaranteed. Applicants for this and a number of other projects will be in competition for studentships funded by the Northern Ireland Department for the Economy (DfE)**
Full eligibility (including residency conditions) and funding information can be viewed via https://www.nidirect.gov.uk/articles/department-economy-studentships
Candidates must possess or expect to obtain, a 2:1 or first-class degree in Chemistry or closely related discipline
Candidates must be available to start the post by October 2024
For more information please contact: Prof. Peter Nockemann (p.nockemann@qub.ac.uk)
Project length: 3 Years full time
Funding body: DfE (competitive against over projects)
Closing date for application: CLOSED
More details and to Apply click Here.
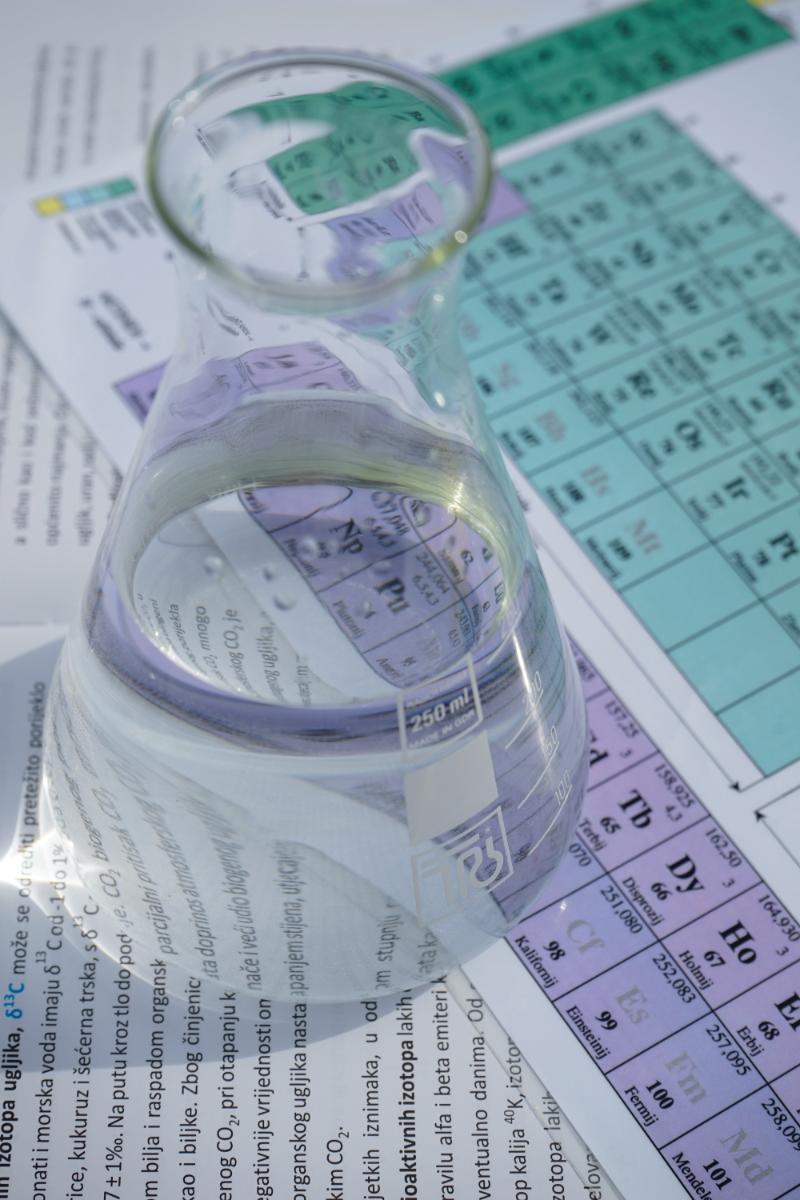
Increased use of renewable energy sources, such as wind or solar, is vital to prevent climate disaster. However, these sources of energy are intermittent (for example, sunlight is only available in the daytime) and non-dispatchable (cannot be shipped). This necessitates an increase in global energy storage capacity of ca. 1000 GWh by 2030 (BloombergNEF). To address seasonal fluctuations in supply and demand of energy, the world is turning to green hydrogen as a potential solution. The most efficient way to convert hydrogen back to electricity is by using a fuel cell, which generates voltage from the separate reactions of hydrogen and oxygen, giving water as the by-product. The most common fuel cell type is the polymer electrolyte fuel cell (PEFC) that uses platinum electrocatalysts and hydrated perfluorinated membranes. However, their commercial adoption is limited by the high cost of these materials and the low tolerance of today’s PEFCs to fuel impurities. Recently, alternative materials, using ionic liquids as components of membranes and catalyst layers, have shown promising results that may unlock greater uptake of this important electrochemical technology.
This PhD project has three main aims: 1) to produce a range of composite membranes (ionomers) from judiciously selected protic ionic liquids and poly(ionic liquids), and characterise their chemical, mechanical, and electrochemical properties; 2) to explore methods for the combination of these ionomers with Pt and non-Pt electrocatalysts and apply electrochemical techniques to probe their viability for use in fuel cells; 3) to produce a membrane-electrode assembly from the most promising candidates for single-cell laboratory testing.
This project sits at the interface between chemistry, materials science, and chemical engineering, taking fundamental insights through to applied solutions. The successful candidate will have experience in chemical synthesis and an interest in energy and sustainability. The PhD candidate will join the School as it develops hydrogen research as a core competence and will have ready access to experts in the field of ionic liquids through affiliation with the QUILL Research Centre.
Funding Information
Full eligibility information and funding information can be viewed via: https://www.ukri.org/councils/esrc/career-and-skills-development/funding-for-postgraduate-training/eligibility-for-studentship-funding/
For more information, please contact: Dr Josh J Bailey (j.bailey@qub.ac.uk)
Project length: 3 Years full time
Funding body: EPSRC
Closing date for application: CLOSED
More details and to Apply click Here.
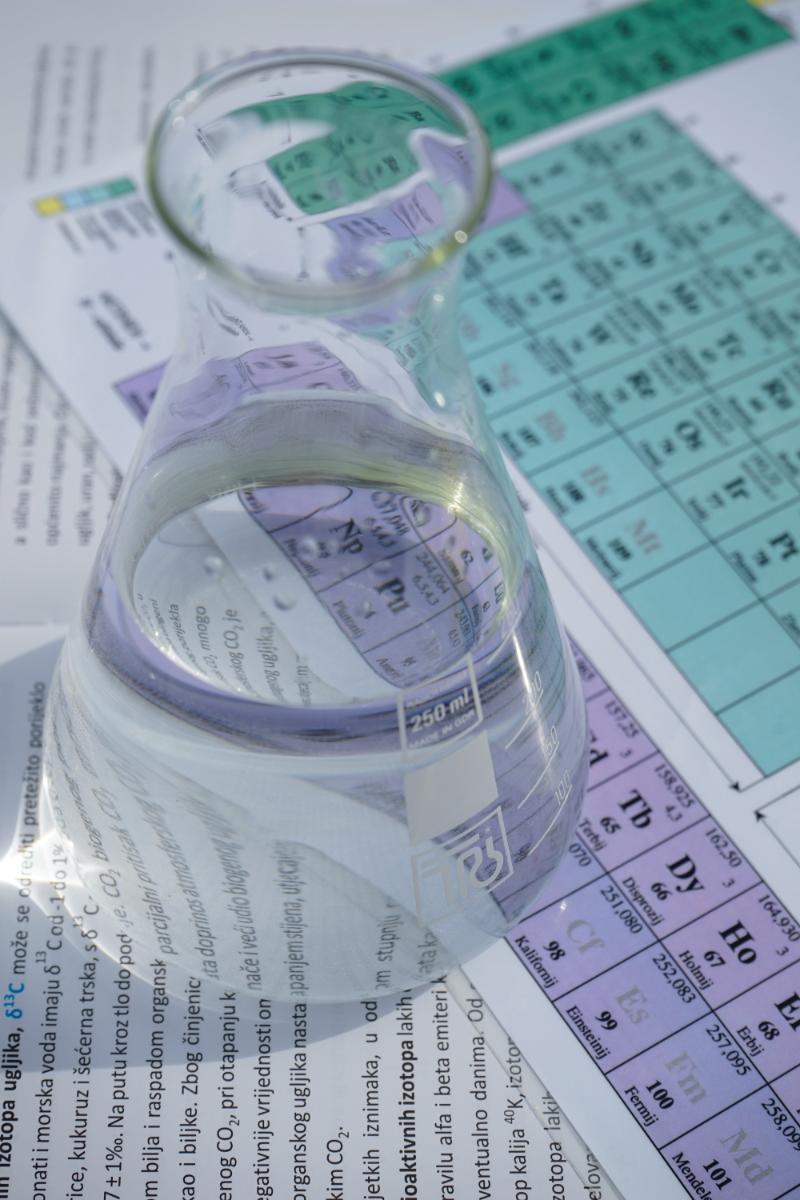
Recycling end-of-life magnets enables recovery of rare earths and transition metals, but also produces metal-rich waste streams containing iron, boron, phosphorus and other by-products. Conventionally, these are disposed of, however transforming them into useful co-products would significantly improve process sustainability and economy. There is an opportunity to develop waste valorisation chemistry that integrates into industrial magnet recycling.
Recycling end-of-life magnets enables recovery of rare earths and transition metals, but also produces metal-rich waste streams containing iron, boron, phosphorus and other by-products. Conventionally, these are disposed of, however transforming them into useful co-products would significantly improve process sustainability and economy. There is an opportunity to develop waste valorisation chemistry that integrates into industrial magnet recycling.
This PhD will conduct a comprehensive investigation into waste valorisation opportunities for an industrial magnet recycling process. After an extensive literature review on techniques and potential by-product applications, the project will focus on developing chemistry for adding value to iron, boron, and phosphorus-rich streams. Firstly, all species in the waste streams will be analysed in terms of average content and chemical speciation.
Then, conventional routes to their selective separations will be tested on real feedstock, but these are known (proof of concept from Ionic Tech) to cause process problems with phase-separation (formation of foams, suspensions, gels). Therefore, this project will develop innovative, selective extractions and precipitations strategies, using semi-sacrificial deep eutectic solvents (DES) to manage and control the phase behaviour. This builds on the proof of concept for the separation of gallium using hydrophobic deep eutectic solvents by the Swadźba-Kwaśny group (IDF submitted).
This project will focus on fundamental inorganic chemistry to selectively convert components of the waste stream into chemicals that have market value as commodity products, such as fertilisers, battery chemicals, catalysts, or pigments. Towards the end of the project, techno-economic assessment will be used to evaluate large-scale feasibility.
In an ideal scenario, we aim to deliver an integrated and optimised magnet recycling flowsheet incorporating new waste valorisation chemistry. This creates a sustainable process recovering both rare earths/metals and transforming residual streams into marketable co-products.
Co-Supervisors: Prof. Peter Nockemann (CCE), Dr Oana Istrate (MAE)
Funding Information
Funding for this project is subject to final contract agreement with the Industry Partner
UK candidates only. Full eligibility (including residency conditions) and funding information can be viewed via https://www.nidirect.gov.uk/articles/department-economy-studentships
Candidates must possess or expect to obtain, a 2:1 or first-class degree in Chemistry or closely related discipline
Candidates must be available to start the post by October 2024
For more information, please contact: Prof Gosia Swadźba-Kwaśny (m.swadzba-kwasny@qub.ac.uk)
Project length: 3 Years full time
Funding body: Industry Partner / DfE funded
Closing date for application: CLOSED
More details and to Apply click Here.
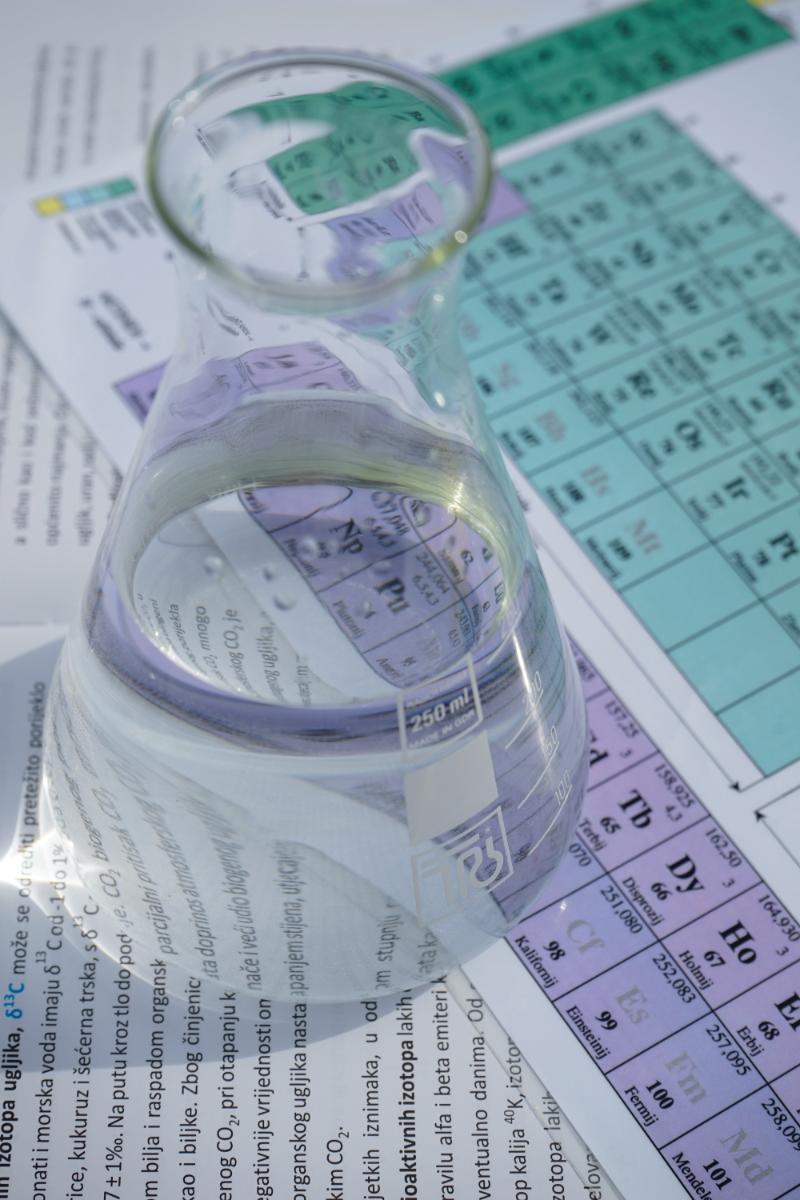
In addressing the environmental consequences linked to carbon emissions and advancing toward a net-zero society, the electrochemical conversion of carbon dioxide (CO2) into value-added chemicals emerges as a promising solution. Despite the substantial potential demonstrated by this approach, a significant hurdle arises from the inadequate solubility of CO2 in conventional liquid electrolytes, restricting the efficiency of CO2 conversion.
This project aims to surmount this obstacle by harnessing the distinctive characteristics of gas-capturing liquids, notably ionic liquids and deep eutectic solvents (DES), employed as electrolytes. Ionic liquids and DES exhibit dual functionality, serving as effective electrolytes while simultaneously capturing and enhancing the solubility of CO2 due to their tailored chemical compositions. The primary focus of the research will be on exploring a diverse range of ionic liquids and DES, which have already demonstrated proficiency as electrolytes in integrating with solid electrocatalytic surfaces for CO2 reduction. The inherent gas uptake capabilities of these liquids are anticipated to not only improve solubility but also enable mixed gas separation, providing a strategic advantage in terms of selectivity for the electrochemical conversion process. Another key advantage of utilising such gas-capturing liquids lies in their ionic composition, which imparts inherent conductivity. This eliminates the need for addition of expensive electrolytic salts to the electrolyte, making the overall electrochemical conversion process more economically viable and sustainable. The project aims to systematically investigate the performance of various gas-capturing liquids in terms of electrochemical efficiency, selectivity, and scalability. By combining electrocatalysts with gas-capturing liquids, this research seeks to establish a novel paradigm for the electrochemical conversion of CO2 to value-added chemicals. The outcomes of this project have the potential to contribute significantly to the development of sustainable technologies, offering a pathway to mitigate the environmental impact of greenhouse gas emissions while simultaneously producing valuable chemical compounds.
Funding Information
** Funding for this project studentship is not guaranteed. Applicants for this and a number of other projects will be in competition for studentships funded by the Northern Ireland Department for the Economy (DfE)**
Full eligibility (including residency conditions) and funding information can be viewed via https://www.nidirect.gov.uk/articles/department-economy-studentships
Candidates must possess or expect to obtain, a 2:1 or first-class degree in Chemistry or closely related discipline
Candidates must be available to start the post by October 2024
For more information, please contact: Dr Paul Kavannagh (p.kavanagh@qub.ac.uk)
Project length: 3 Years full time
Funding body: DfE funded (competitive against over projects)
Closing date for application: CLOSED
More details and to Apply click Here.
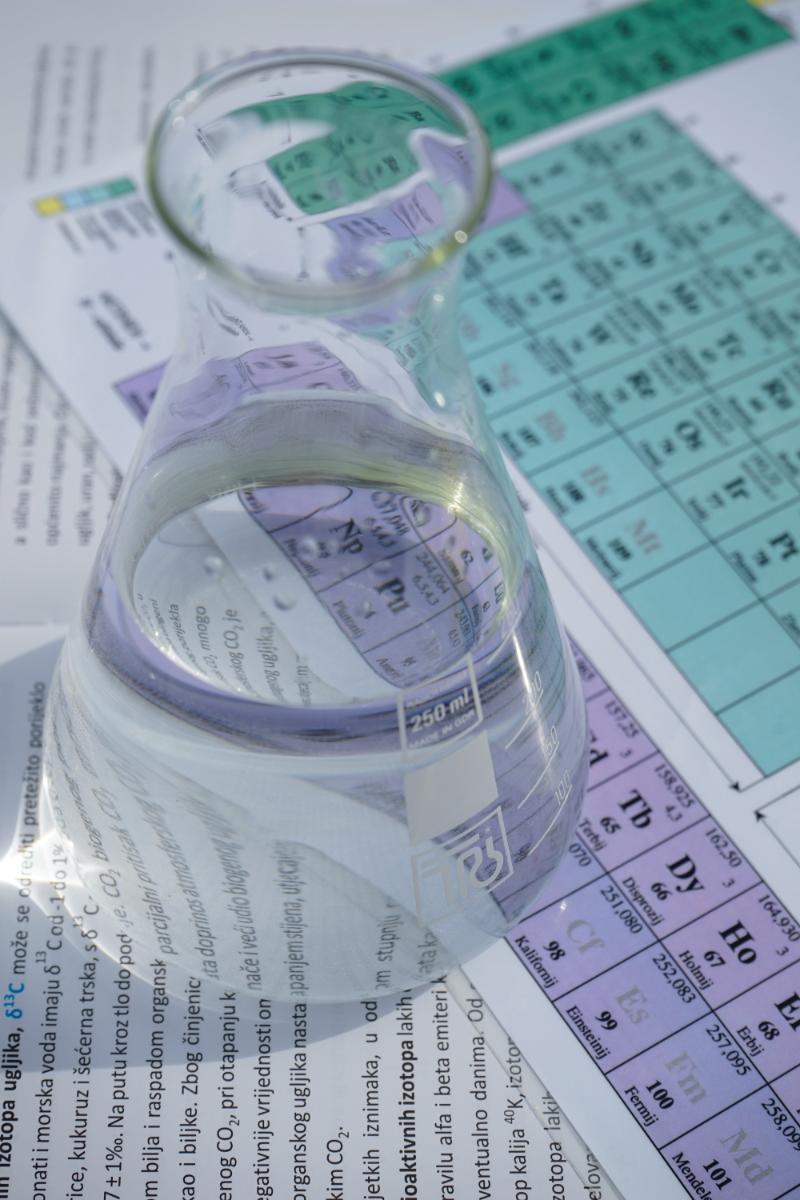
Metal-containing ILs (MILs) built around the MnXm type anion (M = Fe, Co, Ni, Cu, Zn, Al, In, Au; X = Cl, Br, I, SCN), or those with more than one metal, have demonstrated a particularly wide set of properties. Changes in the M or X parts of the anion lead to significant changes in the optical, electronic, magnetic, electrochemical, or catalytic properties of the MIL. Similarly, changes in the cation can, among others, also influence the melting points or viscosities of these MILs. These types of compounds have shown intense colour changes, not only upon exchange of the cation or ligand, but also when placed in contact with certain gases or vapours, providing great opportunities in sensing and gas separation. Despite several gases and vapours being studied/detected with MILs, including VOCs, ammonia, H2S and SO2, there are few studies that investigate the influence of metals, metal combinations, or halides on their structural properties, or the availability of the metal to interact with gases.
This project aims to use small gas molecules to probe the availability and coordination ability of the metal species in the ionic liquid.
This systematic assessment will be performed via headspace gas chromatography and will evaluate the impact of different metal species (mono- and bi-metallic anions), their ligands, the type of cation and whether the IL is in the solid or liquid state, on the coordination properties when exposed to CO2, H2 or ethylene.
Given that MILs have recently demonstrated promising redox activity, small gas probes will also be used to investigate their electrochemical properties. The insights from these results will provide insights into developing optimal MILs as electrolytes for battery applications, gas uptake/sensing systems, and catalysis.
Funding Information
** Funding for this project studentship is not guaranteed. Applicants for this and a number of other projects will be in competition for studentships funded by the Northern Ireland Department for the Economy (DfE)**
Full eligibility (including residency conditions) and funding information can be viewed via https://www.nidirect.gov.uk/articles/department-economy-studentships
Candidates must possess or expect to obtain, a 2:1 or first-class degree in Chemistry or closely related discipline
Candidates must be available to start the post by October 2024
For more information, please contact: Dr Leila Moura (l.moura@qub.ac.uk)
Project length: 3 Years full time
Funding body: DfE funded (competitive against over projects)
Closing date for application: CLOSED
More details and to Apply click Here.
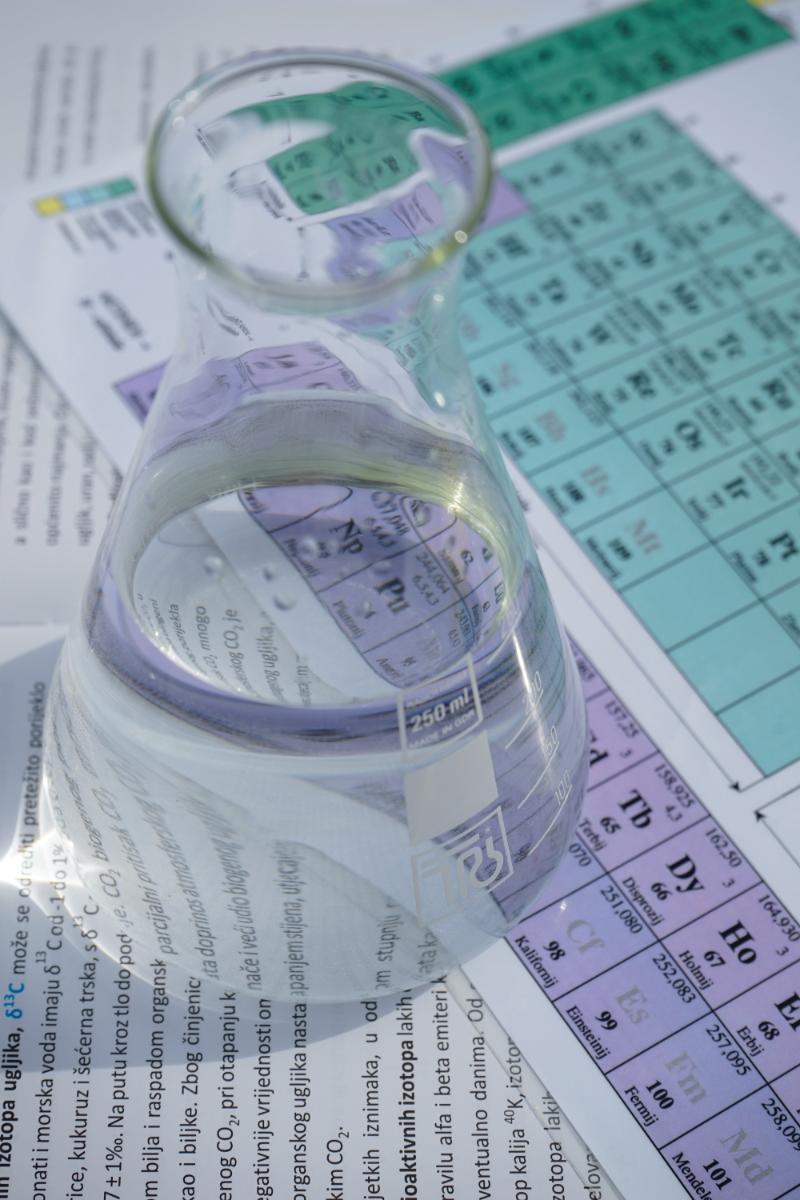
Carbon capture and hydrogen storage are, globally, two of the most discussed research and policy topics, due to their energetic and environmental importance. Material development for these applications follow the lead, with porous materials being one of the most popular and recent options, with publications reaching over 3000/year, with commercially available examples for gas capture, owing to their high sorption capacity and separation abilities. Current microporous materials include metal organic frameworks (MOFs), zeolites, covalent organic frameworks (COFs), activated carbon and few other purely organic materials. These can be difficult to synthesise, obtain sustainably in large scale or recycle, as they are metal-based and often break down with gas stream contaminants.
This project aims to develop resilient organic functionalised microporous polymers (MPs), from sustainable/biobased, waste or commercial sources that can be considered inexpensive, biodegradable, nontoxic, and easy to produce in large scale, making them attractive for commercialisation.
The MPs will be functionalised pre- or post-synthesis to target specific gases, be easily modulated, naturally highly functionalised and less sensitive to contaminants such as water, volatile organic compounds (VOCs), SO2 and H2S. Ultimately, a variety of MPs targeting different impurities will be combined to produce a custom sorbent adaptable to the gas feed composition.
MPs will be commercial and/or based or derived from biomass waste/natural polymers such as cellulose which is cheap, abundant and rich in active functional groups with affinities to CO2 such as (poly)hydroxy, amino, and carboxyl groups. To increase affinity to target gas, functional groups will be chemically introduced by formation of molecularly imprinted polymers, MIPs or frustrated Lewis pairs, FLPs. The pre-assembled polymer mix, imprinting cavities and a supporting matrix are fixed through (hyper)crosslinking reactions, providing (a degree of) size/shape selectivity.
MPs will be screened for gas uptake and selectivity via HS-GC and gas-sorbent interactions will be accessed via liquid and solid-state NMR spectroscopy and thermodynamic properties of solvation. Energetic considerations such as enthalpies of sorption/solvation will feed the design of improved materials by advising modifications in amounts/types of functional groups attracting the gases.
Funding Information
** Funding for this project studentship is not guaranteed. Applicants for this and a number of other projects will be in competition for studentships funded by the Northern Ireland Department for the Economy (DfE)**
Full eligibility (including residency conditions) and funding information can be viewed via https://www.nidirect.gov.uk/articles/department-economy-studentships
Candidates must possess or expect to obtain, a 2:1 or first-class degree in Chemistry or closely related discipline
Candidates must be available to start the post by October 2024
For more information, please contact: Dr Leila Moura (l.moura@qub.ac.uk)
Project length: 3 Years full time
Funding body: DfE funded (competitive against over projects)
Closing date for application: CLOSED
More details and to Apply click Here.
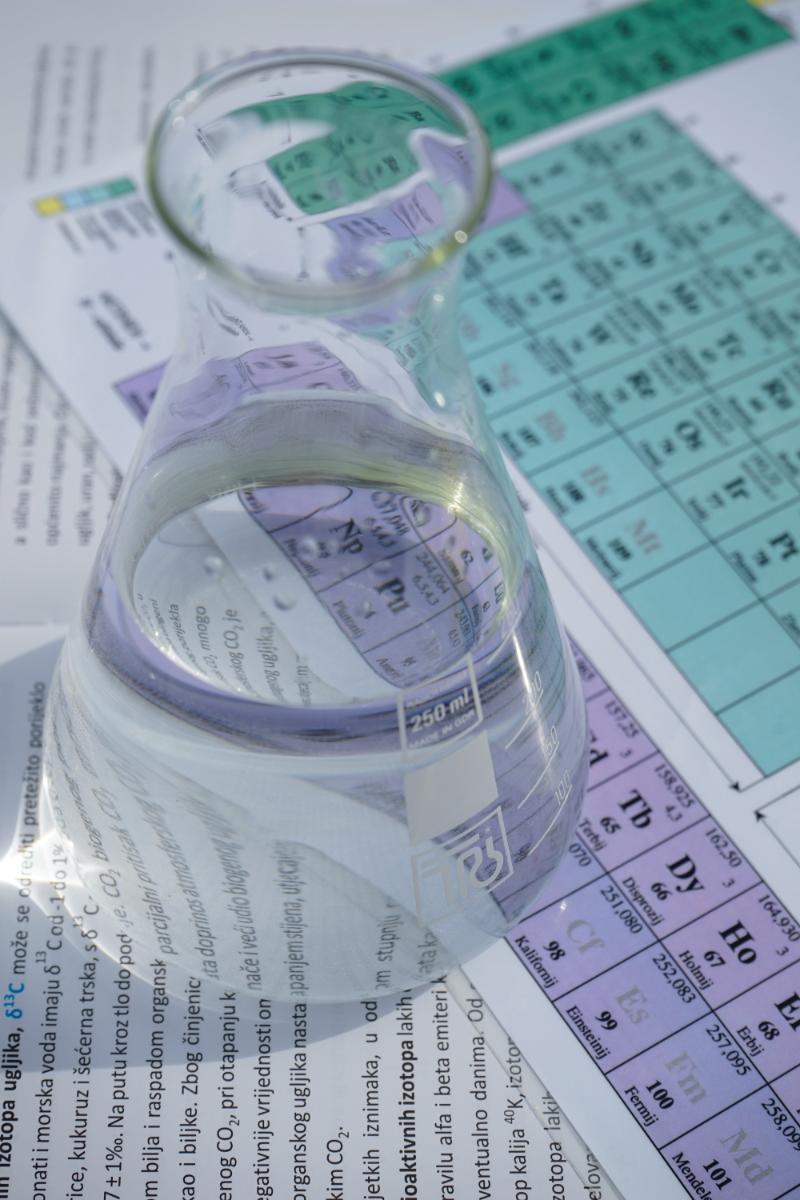
Climate change and growing demand for clean energy are two most impending global challenges that need to be addressed with immediate priority. In this regard, direct capture, and conversion of the CO2 to valuable fuels and chemicals is at the centre stage, because it not only removes the global greenhouse gas from the environment, but also serves as the C1 feedstock for making net zero emission fuels and chemicals. However, activation of CO2 is quite daunting due to its stability and inertness. The conversion of CO2 to C1 fuels such as methanol and dimethyl ether (DME) can be successfully addressed using a combination of catalysis and reaction engineering. At Queen’s, we have developed novel catalytic approaches combined with appropriate reactor design to achieve CO2 transformation into various products such as methanol, formic acid and cyclic carbonates with high selectivity and conversions.
In this project, we will develop an integrated approach using novel catalysts to achieve high conversion and selectivities. Our methodology is as shown in Scheme 1. Direct capture and conversion of CO2 is a two-step sequential process, which requires catalytic hydrogenation of CO2 to methanol in the first step followed by subsequent dehydration of methanol to DME in the second step. Successful production of DME in high yield requires optimisation of two-steps in tandem. In our group, we have recently developed novel catalysts for highly selective conversion of CO2, which is required for the first step of hydrogenation of CO2 [1].
The key scientific objective of the proposed research is to develop prototype catalytic technology for demonstration of direct capture and conversion of CO2 to DME. This objective will be achieved using novel catalysts and reactor design technologies developed in our research groups.
References:
[1] Maddaloni, M.; Centeno-Pedrazo, A.; Avanzi, S.; Mazumdar, N.J.; Manyar, H.; Artioli, N. Novel Ionic Liquid Synthesis of Bimetallic Fe–Ru Catalysts for the Direct Hydrogenation of CO2 to Short Chain Hydrocarbons. Catalysts 2023, 13, 1499. https://doi.org/10.3390/catal13121499
Funding Information
** Funding for this project studentship is not guaranteed. Applicants for this and a number of other projects will be in competition for studentships funded by the Northern Ireland Department for the Economy (DfE)**
Full eligibility (including residency conditions) and funding information can be viewed via https://www.nidirect.gov.uk/articles/department-economy-studentships
Candidates must possess or expect to obtain, a 2:1 or first-class degree in Chemistry, Chemical Engineering or closely related discipline
Candidates must be available to start the post by October 2024
For more information, please contact: Dr Haresh Manyar (h.manyar@qub.ac.uk)
Project length: 3 Years full time
Funding body: DfE funded (competitive against over projects)
Closing date for application: CLOSED
More details and to Apply click Here.
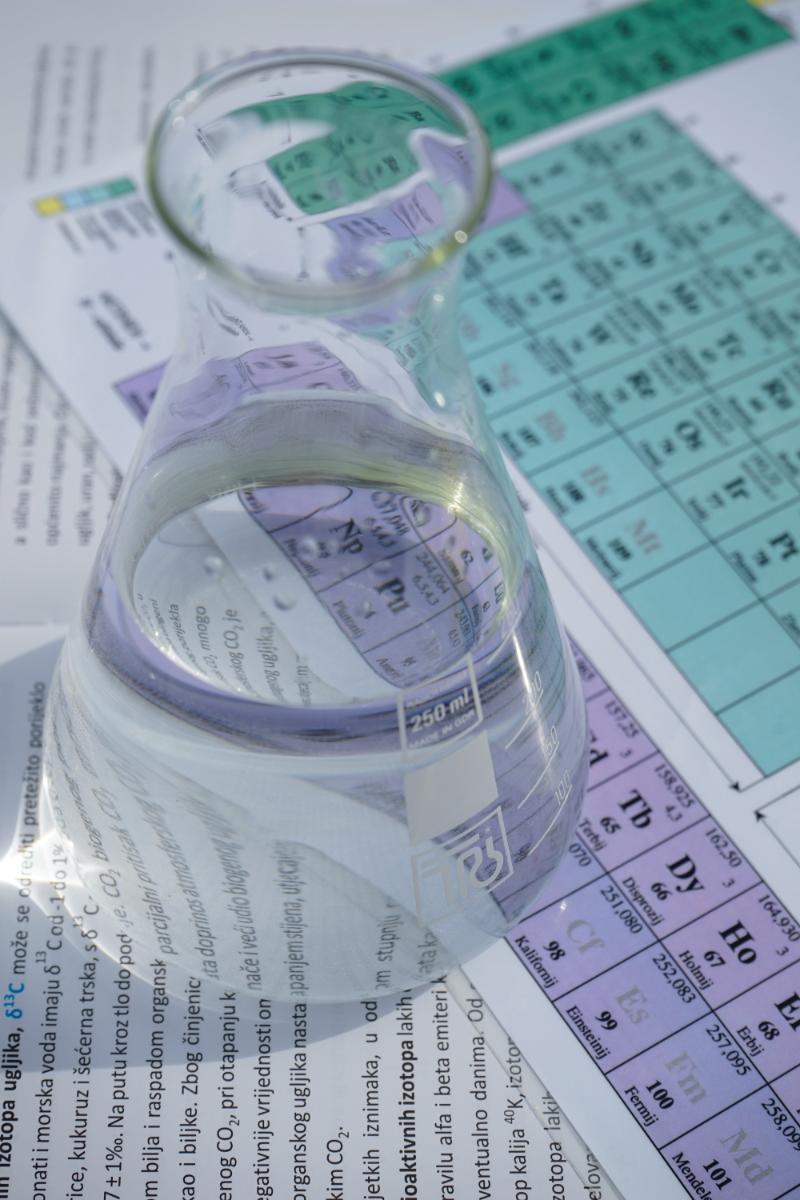
This project is aimed at developing new prototype technology for production of sustainable aviation fuels (SAFs) by using integrated catalytic processes for upgrading volatile fatty acids (VFAs) (Scheme 1). VFAs are abundant feedstock present in the process waters obtained from hydrothermal liquefication of biomass as well as in the form of digestate produced in the anaerobic digestors during biogas production. Conversion of VFAs to SAFs includes three step process, namely ketonisation, aldol condensation or alkylation and hydrogenation (Scheme 1). Recently, we have published the robust catalytic process for the first step of ketonisation using metal oxide catalysts. The process is robust, >96% yield of ketones, with excellent time-on-stream catalyst stability of >100 hrs was achieved. The results have been published [1]. The use water as solvent presents the additional advantage of the spontaneous liquid phase separation of hydrocarbons, improving overall energy efficiency of the process.
In proposed PhD, we will develop the integrated catalytic process for -alkylation & hydrogenation. This PhD would be instrumental for completion of the integrated process, putting QUB at the forefront of sustainable net-zero technologies for production of SAFs.
Reference: [1] Role of sulfation of zirconia catalysts in vapor phase ketonisation of acetic acid, Delarmelina et al., J. of Phys. Chem. C., 2021, doi.org/10.1021/acs.jpcc.1c06920
Funding Information
** Funding for this project studentship is not guaranteed. Applicants for this and a number of other projects will be in competition for studentships funded by the Northern Ireland Department for the Economy (DfE)**
Full eligibility (including residency conditions) and funding information can be viewed via https://www.nidirect.gov.uk/articles/department-economy-studentships
Candidates must possess or expect to obtain, a 2:1 or first-class degree in Chemistry, Chemical Engineering or closely related discipline
Candidates must be available to start the post by October 2024
For more information, please contact: Dr Haresh Manyar (h.manyar@qub.ac.uk)
Project length: 3 Years full time
Funding body: DfE funded (competitive against over projects)
Closing date for application: CLOSED
More details and to Apply click Here.
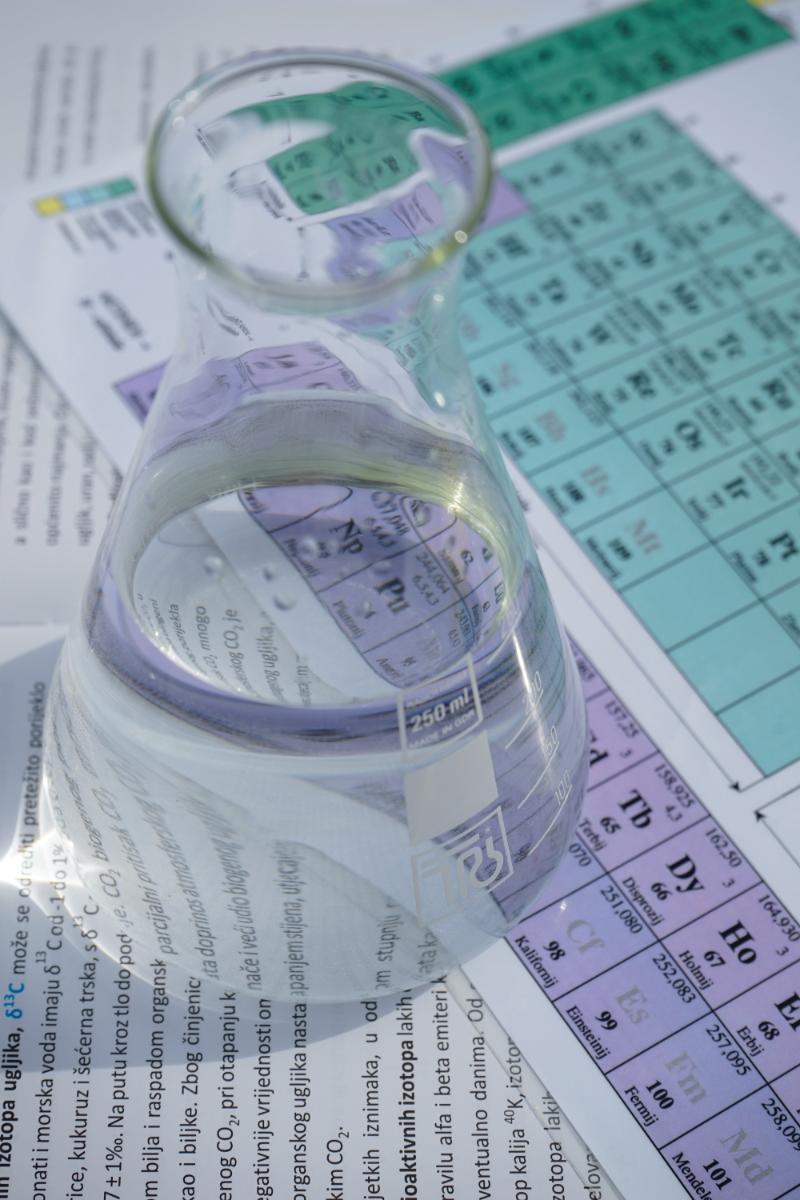
Develop the next generation of flexible strain sensors via an innovative fusion of nanotechnology and 3D printing. Formulate high-performance polymer nanocomposites filled with carbon nanotubes or graphene. Optimise the dispersion and orientation of the nanofiller and develop materials that can transduce mechanical strains into precise electrical signals. Through hands-on 3D printing, give physical shape to your material innovations. Validate sensor performance for wearable and soft robotics applications. Acquire broadly applicable skills in an emerging field with tremendous technological potential at the vibrant intersection of materials science and mechanical engineering.
Flexible strain sensors made from polymer nanocomposites can be used for soft robotics and for monitor human activities through wearable devices. By transducing mechanical deformations into electrical signals, they can measure respiration rate, joint motions, etc. These capacitive, resistive, or piezoelectric devices capitalise on polymers’ stretchability combined with nanofillers’ strength. Additive manufacturing through 3D printing facilitates shaping novel materials with heightened sensor sensitivity.
By incorporating nanoscale materials like carbon nanotubes (CNTs) or graphene into a stretchable polymer matrix, the resulting nanocomposite exhibits both the durability and conductivity required for strain sensing. The polymer matrix provides flexibility, stretchability, and durability, while the embedded nanomaterial, e.g., carbon nanotubes or graphene enable sensitive transduction of mechanical deformations into electrical resistance changes. By tuning the nanofiller morphology, dispersion, orientation and polymer-filler interfacial interactions, large gauge factors over a wide strain range can be achieved.
This project aims to synthesise superior composites by reinforcing polymers with conductive nanotubes or graphene, then 3D print soft sensors. With augmented electromechanical response, these sensors hold promise for artificial muscles, robotics, and other applications at the intersection of nanotechnology and additive manufacturing. The overarching goal of this project is to formulate novel CNT- and graphene-based polymer nanocomposites, optimise their electromechanical response, and fabricate functional prototypes via 3D printing.
The key transferable skills that will be developed during this PhD project will be in the areas of:
Interdisciplinary research, industry collaboration, specialist techniques, communication, teamwork.
Funding Information
UK studentships - cover tuition fees and include a maintenance stipend of £18,622 per annum, together representing an investment in your education of more than £70,000.
A UK studentship is open to UK and ROI nationals, and to EU nationals with settled status in the UK, subject to meeting specific nationality and residency criteria.
DfE studentship eligibility information can be viewed at: https://www.economy-ni.gov.uk/publications/student-finance-postgraduate-studentships-terms-and-conditions
For more information, please contact: Dr Oana Istrate (O.Istrate@qub.ac.uk)
Project length: 3 Years full time
Funding body: DfE funded
Closing date for application: 4 March 2024
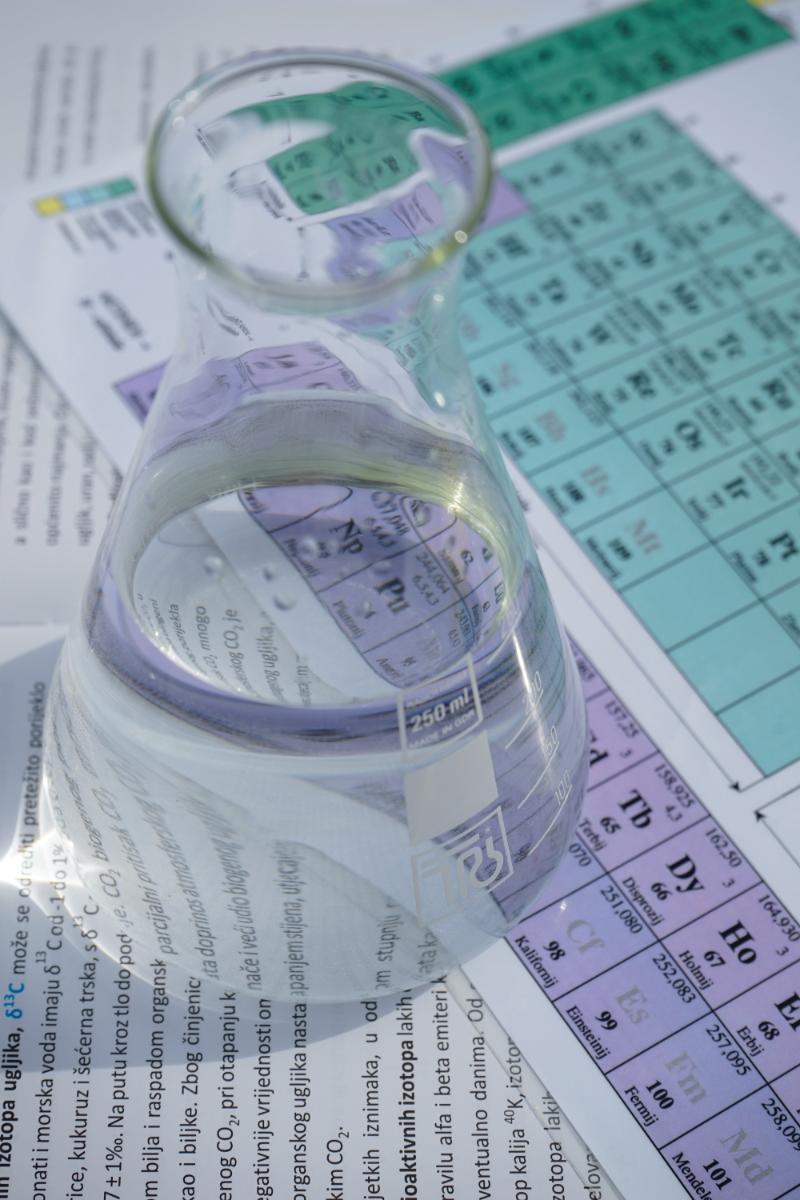
Efficient demagnetisation of end-of-life permanent magnets is critical for recycling rare earths and metals, but current industrial processes use energy-intensive thermal treatment. This PhD project will investigate optimised, sustainable demagnetisation methods using multiphysics simulations and lab experiments with different heat sources like infrared, induction furnaces, and microwaves. By modeling heat transfer and magnetic domain evolution in COMSOL, we will predict optimal configurations and parameters for thermal demagnetisation. Validations will be through demagnetisation experiments, with magnetic properties evaluated via SQUID magnetometry. We aim to deliver optimised protocols to minimise energy use while maintaining material integrity, advancing physics and engineering principles for sustainable magnet recycling.
Efficient demagnetisation is a critical first step in recycling end-of-life permanent magnets to enable separation and recovery of rare earths and valuable metals. However, current industrial processes use energy-intensive thermal treatment to destroy magnetic domains. There is need to develop optimised, sustainable demagnetization methods.
This PhD project will investigate optimised demagnetisation of end-of-life magnets using different heat sources, such as infrared, induction furnaces, or microwave heating. The project will model heat transfer and magnetic domain evolution using multiphysics simulation software like COMSOL. Different heating methods and configurations will be simulated to predict optimal thermal demagnetisation. Validation will be through lab-scale demagnetisation experiments with magnetic properties evaluated using sensitive SQUID magnetometry. The effect of heating parameters on microstructure will also be analysed.
The goal is to develop an in-depth understanding of efficient, controllable demagnetisation to minimise energy use and maintain material integrity in an industrial recycling context. By combining multiphysics modelling with tailored experiments, we aim to deliver optimised demagnetisation protocols along with new insights into thermal magnetic transition mechanisms. The project will have strong emphasis on physics and engineering principles for sustainable recycling.
The key transferable skills that will be developed during this PhD project will be in the areas of:
Interdisciplinary research, industry collaboration, specialist techniques, communication, teamwork.
Funding Information
UK studentships - cover tuition fees and include a maintenance stipend of £18,622 per annum, together representing an investment in your education of more than £70,000.
A UK studentship is open to UK and ROI nationals, and to EU nationals with settled status in the UK, subject to meeting specific nationality and residency criteria.
DfE studentship eligibility information can be viewed at: https://www.economy-ni.gov.uk/publications/student-finance-postgraduate-studentships-terms-and-conditions
For more information, please contact: Dr Oana Istrate (O.Istrate@qub.ac.uk)
Project length: 3 Years full time
Funding body: DfE funded
Closing date for application: 4 March 2024
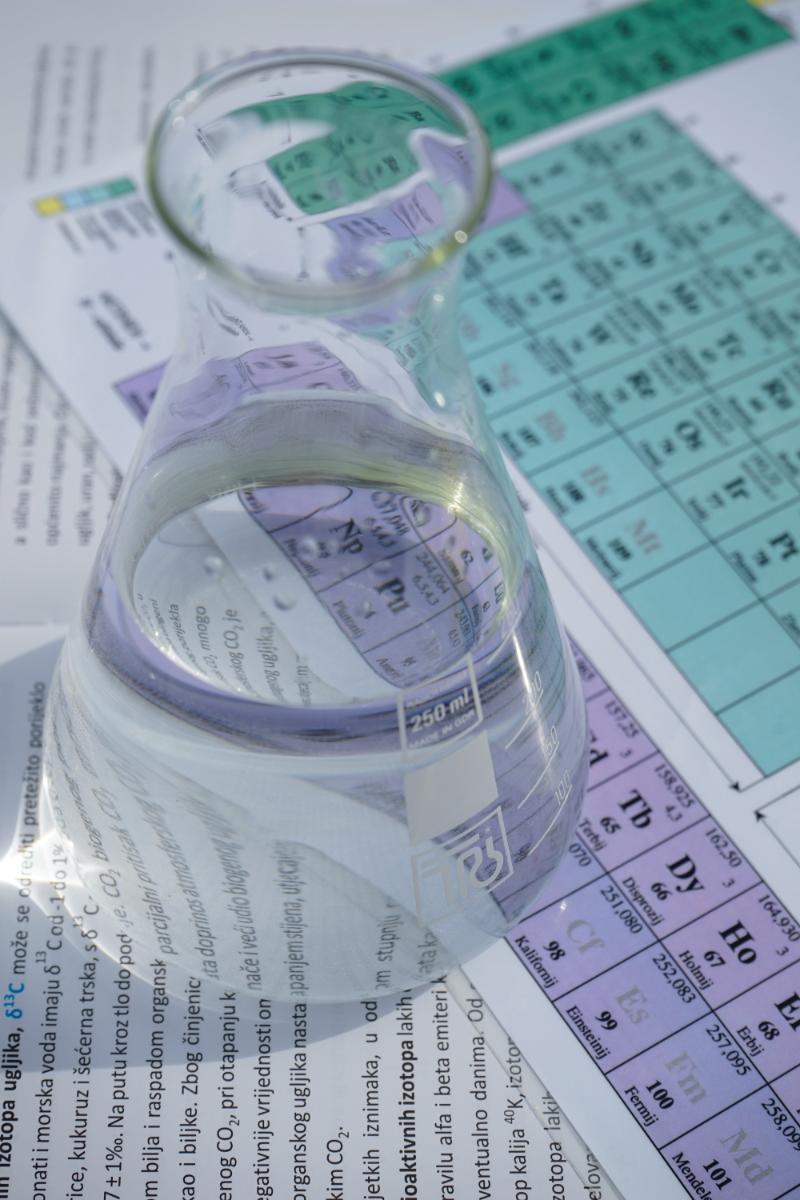
When implanted in the human body or exposed to biological fluids, biomedical devices such as implants, catheters, and infusion lines often encounter surface biofouling. This phenomenon arises from the adsorption of proteins and lipids, leading to subsequent bacterial colonization. It is estimated that approximately 65% of implanted devices trigger infections and related complications due to biofilm formation.
This project aims to address the challenge of biofouling through the development of innovative surface coatings. Our focus will be on leveraging recently developed functional entities within our group, namely multizwitterionic compounds and hybrid zwitterionic salts that uniquely combine zwitterionic and cationic entities within a single molecule. Both types of molecular motifs are characterised by enhanced hydration compared to single zwitterionic functionality. This enhanced hydration forms a protective water layer, acting as a barrier against protein attachment to the medical device's surface.
Antifouling Coating Design: The proposed antifouling coating will be applied to relevant metallic and polymeric surfaces and tested for protein adsorption and antimicrobial activity. Coatings functionalized with multizwitterionic compounds offer the potential to mitigate the issue of low grafting density of functional units, while on the other hand, coatings based on hybrid zwitterionic-cationic entities will be designed to provide a synergistic ‘layer by layer’ resistance to protein attachment along with antimicrobial activity.
Self-Assembly: The synthesised multizwitterionic and hybrid zwitterionic salts, being intrinsically amphiphilic, are expected to aggregate in aqueous solutions, resulting in diverse self-assembly structures having zwitterionic interface as protection against protein opsonization. The study will explore their hierarchical organisation, potentially extending the application to areas such as drug delivery and medical imaging. This aspect of the research will involve neutron-scattering data collection and analysis, and molecular modelling.
Interdisciplinary Approach and Broader Impact: The PhD program will benefit from established intra- and inter-departmental collaborations. The candidate will acquire experience in design, synthesis of organic molecules, and utilising a wide range of analytical and spectroscopic techniques, including UV and fluorescence spectroscopy, NMR, DLS, DSC, TGA, QCM, and microscopic imaging. Beyond the direct aims of the project, the materials, methods, and understanding developed through this research are expected to have broader effects and applications. The project offers a comprehensive experience encompassing various aspects of material science, chemistry, and microbiology including bacterial and cell culture assays.
Funding Information
** Funding for this project studentship is not guaranteed. Applicants for this and a number of other projects will be in competition for studentships funded by the Northern Ireland Department for the Economy (DfE)**
Full eligibility (including residency conditions) and funding information can be viewed via https://www.nidirect.gov.uk/articles/department-economy-studentships
Candidates must possess or expect to obtain, a 2:1 or first-class degree in Chemistry, Chemical Engineering or closely related discipline
Candidates must be available to start the post by October 2024
For more information, please contact: Dr Marijana Blesic (m.blesic@qub.ac.uk)
Project length: 3 Years full time
Funding body: DfE funded (competitive against over projects)
Closing date for application: CLOSED
More details and to Apply click Here.
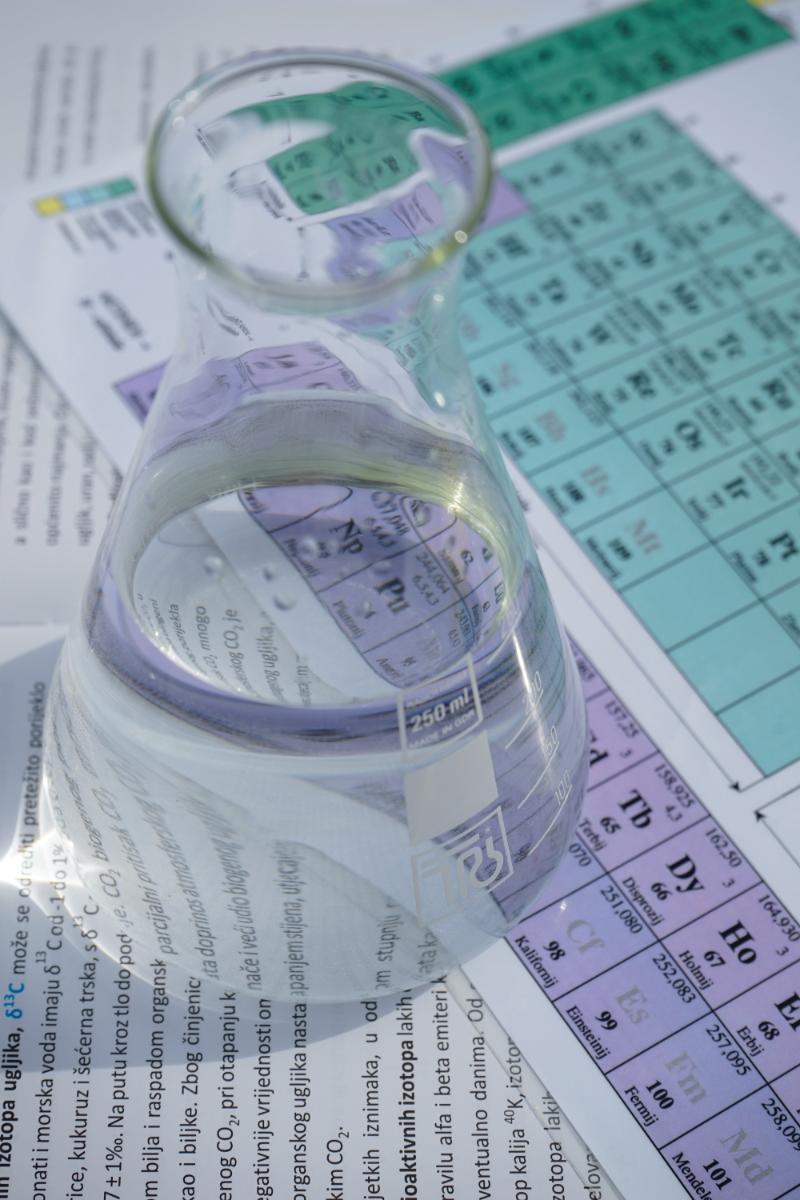
The methods of synthesis of active pharmaceutical ingredients have been undergoing a revolution over the past decade driven by biocatalysis. Biocatalytic methods have become the most efficient method of functional group transformation, completely solving chemo and stereoselectivity issues encountered by purely chemical routes. Project partners Almac have been at the forefront of this change. Proteins still have a drawback however, their stability is often lower in comparison with chemical reagents, particularly when exposed to organic solvents and reagents
In a 4-year-old collaboration QUB and Almac have been looking at the application of entrapment methods1 to protect the protein from poisoning and denaturing. Enzymes are entrapped into ionic liquid gels.2 Ionic liquids are used to tune the environment around the enzyme and to ensure facile diffusion of the substrate and product.3 The result is the production of immobilized biocatalysts that are simple to operate, separate and recycle.4,5
The research is at an exciting stage, and a range of materials and ionic liquids have been shown to support enzymatic activity and recycling. Moving forward, 21st century methods will be explored such as flow biocatalysis, multi-enzyme cascades and 3D printing. It is anticipated that the main enzyme classes studied will be transaminases and ketone reductases.
The well-established collaboration with Almac provides a number of benefits to the student, including placements to receive first-hand experience of researching in industrial laboratories, frequent meetings with industrial partners, advice on analytical and reaction methods, and receipt of libraries of industrial biocatalysts. Plus, and perhaps most excitingly, the possibility to transfer your results directly into the pharmaceutical industry.
[1] Enzyme entrapment, biocatalyst immobilization without covalent attachment, H. T. Imam, P. C. Marr, A. C.Marr, Green Chem., Green Chem., 2021, 23, 4980–5005.
[2] Ionic liquid gel materials: applications in green and sustainable chemistry, P. C. Marr, A. C. Marr, Green Chem., 2016, 18, 105–128.
[3] Applications of Ionic Liquids in Whole-Cell and Isolated Enzyme Biocatalysis, H.T. Imam, V. Krasňan, M. Rebroš, A. C. Marr, Molecules, 2021, 26, 4791.
[4] Supramolecular Ionic Liquid Gels for Enzyme Entrapment, H. T. Imam, K. Hill, A. Reid, S. Mix, P. C. Marr, A. C. Marr, ACS Sustainable Chem. Eng. 2023, 11, 6829–6837.
[5] Entrapment in HydrIL Gels: Hydro-Ionic Liquid Polymer Gels for Enzyme Immobilization, J. A. Pérez Tomás, R. Brucato, P. Griffin, J. Kostal, G. Brown, S. Mix, Stefan, P. C. Marr, A. C. Marr, SSRN: http://dx.doi.org/10.2139/ssrn.4636612
Funding Information
** Funding for this project studentship is not guaranteed. Applicants for this and a number of other projects will be in competition for studentships funded by the Northern Ireland Department for the Economy (DfE)**
Full eligibility (including residency conditions) and funding information can be viewed via https://www.nidirect.gov.uk/articles/department-economy-studentships
Candidates must possess or expect to obtain, a 2:1 or first-class degree in Chemistry or closely related discipline
Candidates must be available to start the post by October 2024
For more information, please contact: Dr Andrew Marr (a.marr@qub.ac.uk)
Project length: 3 Years full time
Funding body: DfE funded (competitive against over projects)
Closing date for application: CLOSED
More details and to Apply click Here.
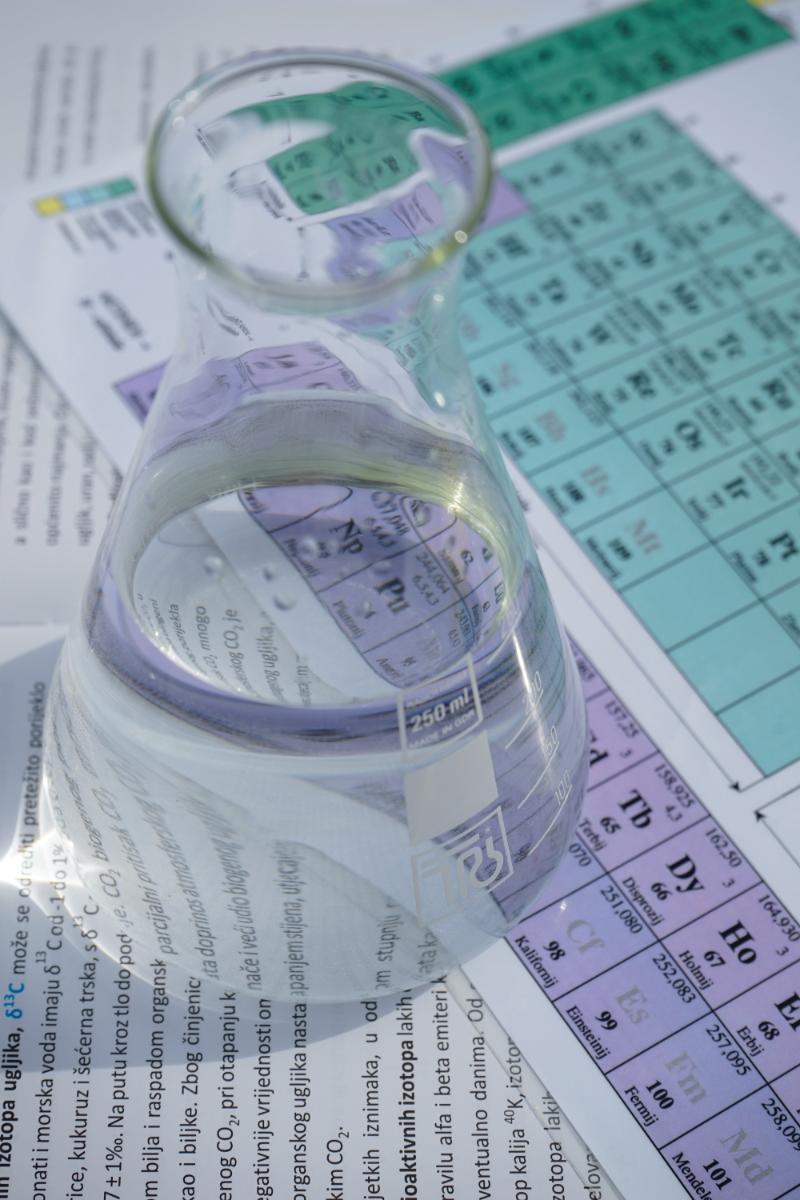
Critical Elements are important for the economy, in particular for modern technologies, but their secure supply is at risk (for example, because they are sourced from a politically volatile region). For such elements, it is very important to develop closed recycling loops, in which waste components are processed to reclaim critical elements, that are subsequently reused. An example of such strategically important components, enabling the transition to renewable energy sources, are strong and lightweight Rare Earth Permanent Magnets (REPM), found in electric vehicles motors and off-shore wind turbines. Manufacturing of such magnets is reliant on rare earth elements, such as neodymium.
Funding Information
This funded project is open only to UK students or ROI students that meet the eligibility criteria. Full eligibility (including residency conditions) and funding information can be viewed via View Website
Candidates must possess or expect to obtain, a 2:1 or first-class degree in Chemistry, Chemical Engineering or closely related discipline
Candidates must be available to start the post by October 2024
For more information, please contact: Prof. Gosia Swadzba-Kwasny (m.swadzba-kwasny@qub.ac.uk)
Project length: 3.5 Years full time
Funding body: EPSRC funded
Closing date for application: 21st June 2024
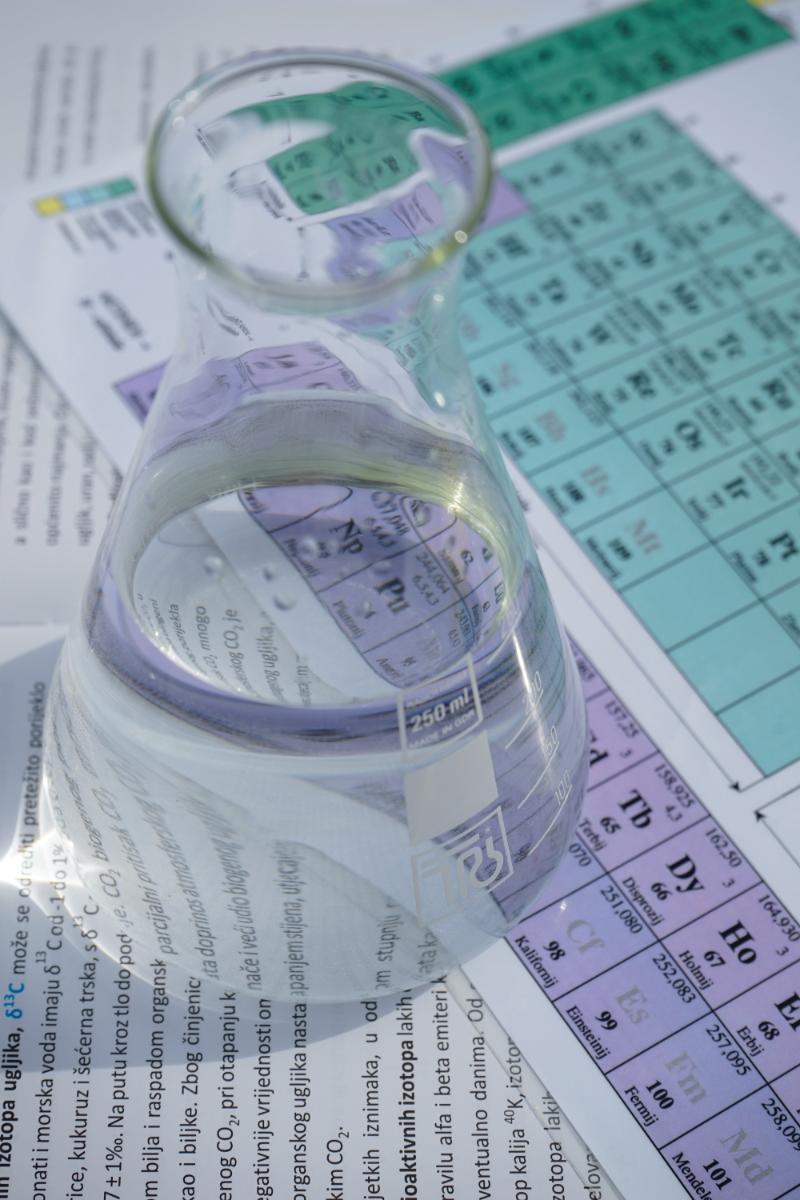
Critical Elements are the elements that are important for the economy, in particular for modern technologies, but their secure supply is at risk (for example, because they are sourced from a politically volatile region). For such elements, it is very important to develop closed recycling loops, in which waste components are processed to reclaim critical elements, that are subsequently reused. An example of such strategically important components, enabling the transition to renewable energy sources, are Rare Earth Permanent Magnets, used in the motors of electric vehicles and wind turbines. Manufacturing of such magnets is reliant on rare earth elements, such as neodymium, dysprosium and terbium.
Funding Information
This funded project is open only to UK students or ROI students that meet the eligibility criteria. Full eligibility (including residency conditions) and funding information can be viewed via View Website
Candidates must possess or expect to obtain, a 2:1 or first-class degree in Chemistry, Chemical Engineering or closely related discipline
Candidates must be available to start the post by October 2024
For more information, please contact: Dr Oana Istrate (o.istrate@qub.ac.uk)
Project length: 3.5 Years full time
Funding body: EPSRC funded
Closing date for application: 21st June 2024
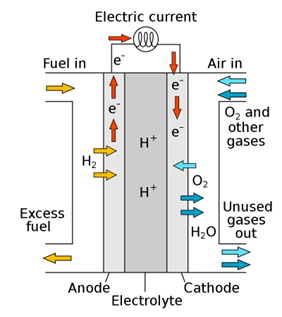
Faced with climate change, renewable energy generation is increasingly rapidly, and so is the need for energy storage, given its intermittency. Fuel cells are important energy conversion devices to sustainably produce electricity from hydrogen, a fuel that is being produced more sustainably as the world decarbonises. Polymer electrolyte fuel cells (PEFC) are the most commercialised variant of this technology, with advantages including high power density, fast start-up, and manufacturing maturity. However, on top of high costs, current PEFC membranes show high gas crossover and restricted operation in dry conditions.
This project looks at fabricating alternative membranes from the polymerisation of protic-ionic-liquid-based monomers and characterising their resultant properties to understand their potential for use in anhydrous PEFCs. The work will entail benchtop synthesis of polymeric materials and optimising the casting of these materials into thin membranes. A wide suite of analytical techniques is available in the department for characterisation of these membranes, and judicious selection of the appropriate tools will be made to understand the differences in their properties. The most promising candidate will be used as an ionomer material in formulated inks and sprayed onto the membrane material, thus preparing novel membrane-electrode-assemblies (MEA) for use in fuel cells.
Deadline: Wednesday 19th June
Anticipated outcome:
Data collected during this project will form part of an application for an EPSRC NIA grant.
Duration (up to 12 weeks) and orientational dates:
10-week project with starting date on 24th June and ending in 30th of August
For more detail and to apply, please contact directly Dr Josh BAiley (j.bailey@qub.ac.uk)