Our Research Scientist's Photography Gallery
Here are some photographs of beautiful STEM from scientists and mathematicians in our department.
Dr. Charlotte Palmer (and Omega TDYNO team) - Centre for Plasma Physics
At the centre of a 6 m diameter metal sphere, 20 laser beams hit two foils that are each thinner than a human hair. The energy in the laser beams is enough to tear apart the atoms in the foils and create plasma, a glowing material that makes up > 99% of the visible universe (the stars, the galaxies and the space between!). In this plasma we can model the processes happening in extreme environments like those around exploded stars. Poking in from the walls of the chamber, lots of diagnostics carefully watch the central plasma and help us learn about these far away events. Here, we are measuring the way that the plasma moves and the magnetic field grows with the goal of exploring whether high energy particles arriving at Earth (cosmic rays) could be accelerated in the exploded plasma around dead stars.
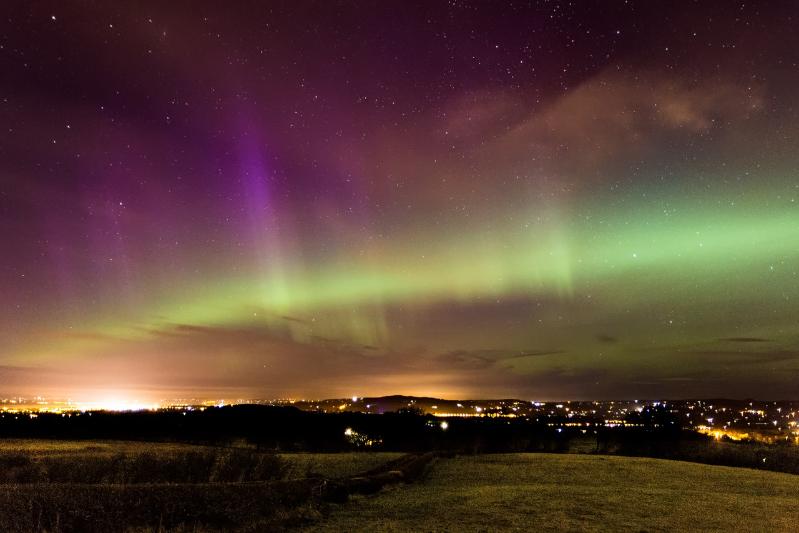
Prof. Alan Fitzsimmons - Astrophysics Research Centre
Looking over the hills of Antrim, the Northern Lights shine down, caused by electrons blown out by the Sun in a "Solar Wind". Astronomers at QUB study our Sun, including the Solar Wind. As the electrons in the Solar Wind are charged, they are deflected by our planets' magnetic field. But sometimes they can get through and enter our atmosphere. Guided by the Earth’s magnetic field, they hit atoms in the atmosphere and make it glow. Green light comes from oxygen atoms in the thin air 100km to 150km above us, while the purple-red colour comes from oxygen in the even thinner atmosphere between there and space. The colours are dictated by quantum physics. The orange and white glow from streetlights and buildings prevents people seeing the display above them. Now the Sun is in a low activity state, and sightings of the Northern Lights are rare. Over the next 5 years the Sun will start ejecting more particles, and more people will once again see this sight from our countryside.
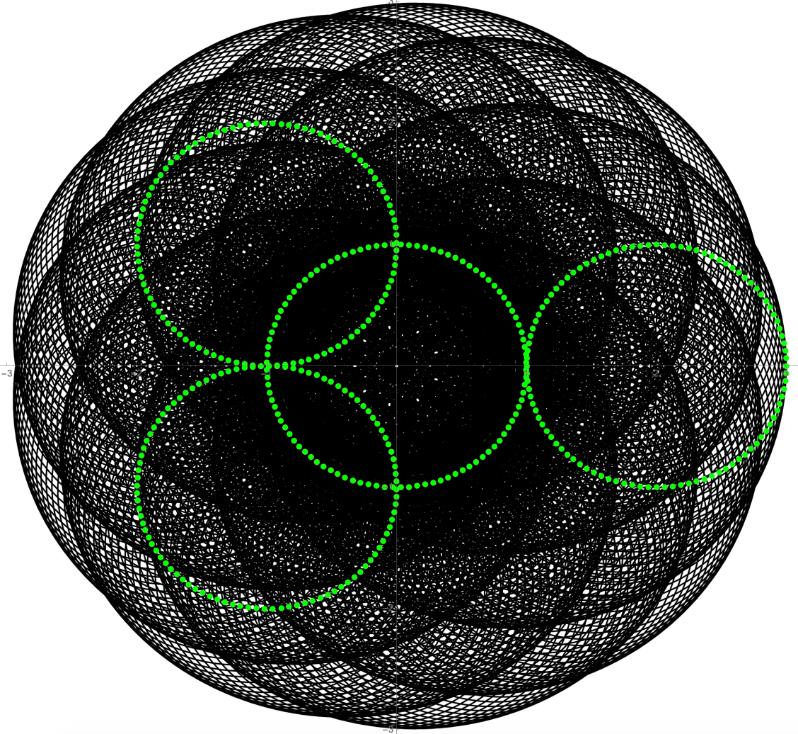
Dr Florian Pausinger - Mathematical Sciences Research Centre
This seemingly highly symmetric black lump is the graph of a well defined closed curve and the green dots mark very specific locations on this graph. This image is an illustration of a mathematician’s daily task. In my research I am confronted with complex structures that can often be understood once hidden structures are uncovered. The answers can sometimes be surprising. In the case of the image the green dots prove that the underlying graph is not symmetric at all — in contrast to an intuitive first guess.
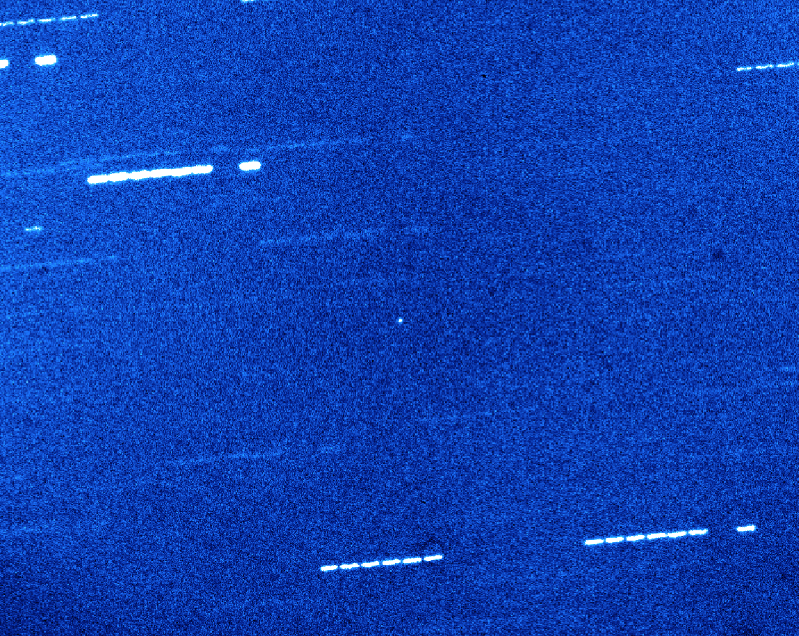
Prof. Alan Fitzsimmons - Astrophysics Research Centre
The small white dot in the centre of the image was the first object discovered visiting our Sun from another Solar system. Found by a telescope in Hawai'i as it passed our planet in October 2017, and travelling too fast for our star’s gravity to hold onto it, astronomers only had a few days to study it before became too far away and too faint. We found it was probably only 200 metres in length, elongated and tumbling as it passed by us, could have ice below the surface, and looked similar to some objects that orbit our Sun. It was named 'Oumuamua, a Hawai'ian word meaning "first messenger from afar". This picture was taken by us using a large telescope in the Canary Islands on 29th October 2017, following 'Oumuamua as it headed back out among the stars. The white streaks and dashes are normal background stars that have moved across the image, while we followed 'Oumuamua across the sky as it departed.
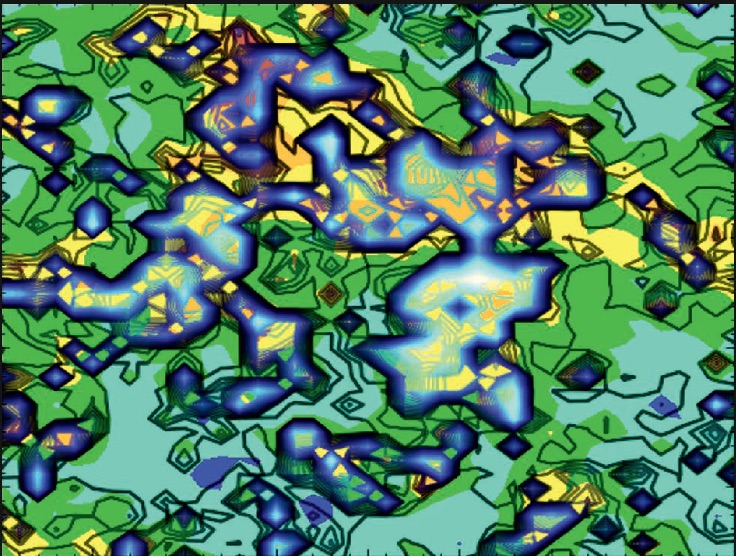
Dr. Anna Zhigun and collaborators (Dr. Sandesh Hiremath, Dr. Stefanie Sonner, Prof. Christina Surulescu) - Mathematical Sciences Research Centre
Cancer cells are cells which no longer ’obey’ the body because their ’memory’ (genotype) is broken. They proliferate relentlessly and spread out. For this activity, they require a lot of resources, particularly glucose. Digesting this sugar, cells create very acidic regions around them. This is harmful for normal body cells, and helps cancer cells by enhancing their migration and making therapies less effective. Despite the available screening, it is difficult to capture the exact contours of a tumour. Unnoticed areas can become sources of tumour relapse. Mathematical modelling can help predict the tumour spread speed and its contours. We developed a mathematical model for acid-mediated tumour invasion. The image shows a simulated tumour and acidity pattern (contour lines and colour patches, respectively) from our model. Lighter colours: high densities of cells and acid concentrations, Darker colours: low densities and concentrations.
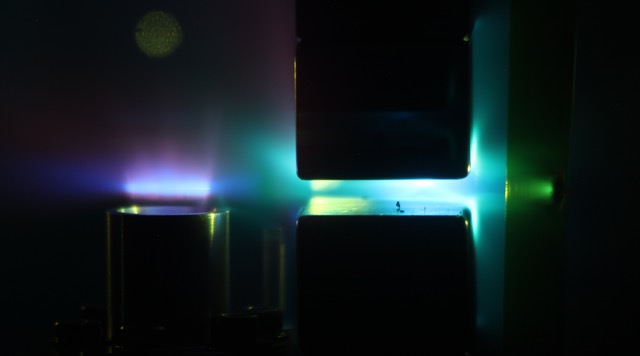
Dr. Matthew Streeter and collaborators - Centre for Plasma Physics
This photo is from an experiment using one of the most powerful lasers in the world at the Central Laser Facility in the UK. The purple channel to the left is glow from a column of hot plasma created by the passage of a focused laser pulse through a gas jet which flows up from the nozzle below. The laser is so intense that it rips apart the atoms of the gas generating a plasma with separate positively charged nuclei and negatively charged electrons. Some electrons are pulled along in the wake of the laser pulse and accelerated to 99.999997% of the speed of light in just 2 centimetres. The laser and electrons then pass through a strong magnet, where they are separated before the laser hits a plastic tape to the right of the image. Blue and green emission is seen as the material of the magnet and tape is also turned to plasma by the still highly intense laser pulse. These plasma accelerators are used to study atomic processes which occur in less than one trillionth of a second.
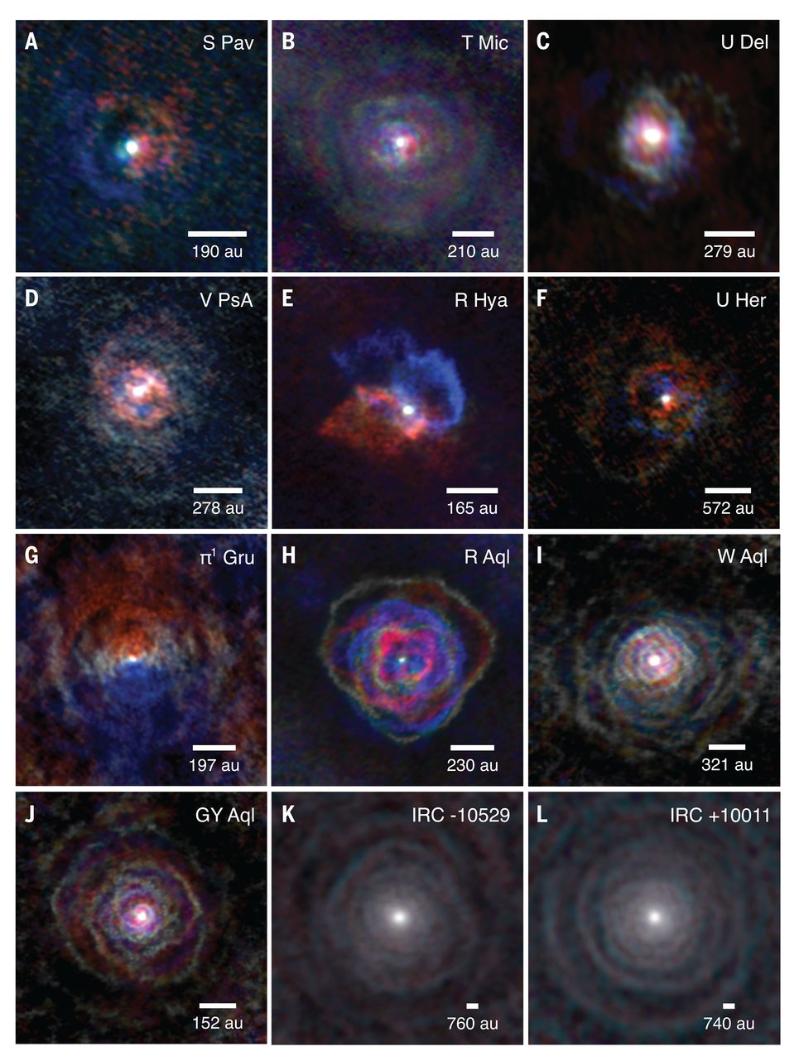
Professor Tom Millar - Astrophysics Research Centre
A century-old puzzle in astronomy is why planetary nebulae have an extraordinary range of shapes, with some symmetry, but are almost never round. How do their shapes arise from the evolution of stars that are spherical? Here are images of the winds from old stars that will evolve into planetary nebulae. They are taken at a wavelength of 2.6mm and trace the motion of CO molecules that flow out from the stellar surfaces with radii of 2-5 astronomical units (au). Red is material flowing away, blue is toward us and white is in the plane of the sky. All the winds are non-radial and are disturbed from radial motion by a close-in orbiting companion, an unseen star or a planet. The properties of the star-companion system determines the shape of the wind and ultimately of the planetary nebula that it will form. These images are part of the ATOMIUM Project using ALMA, 66 radio telescopes situated at an altitude of 5000m in the Atacama desert in northern Chile.
Chris McCooey, Dr Todorov and Dr Dundas - Atomistic Simulation Centre
Nanowires are the smallest wires in existence, making it possible to fit a hundred thousand of them along the width of a human hair. Despite their size, they can transport current densities many orders of magnitude greater than in an ordinary wire in your home. Their amazing properties would help make electronics smaller and more efficient. However, the large current densities that pass through can violently rip the nanowire apart. Our research focuses on making nanowire more stable and deepening our understanding of the physics at play. Here, we show the curl of the electron wind force in a simple constriction at two different scales. Regions in red or blue show areas in then anowire that could potentially break from the action of the oncoming current. The simple constriction creates stresses further on down the wire that are quasi-periodic.
Dr Ryan Milligan - Astrophysics Research Centre
A total solar eclipse is the most awe-inspiring sight in nature. Moments before totally, as the last of the sunlight streams between the lunar mountains, you get a fleeting glimpse of the chromosphere (literally: colour-sphere), a thin layer of the Sun’s atmosphere comprised of excited hydrogen atoms that give it a distinct red colour. My research focuses on solar flares, the largest explosions in the solar system, and the bulk of a flare’s energy emanates from the chromosphere. So studying this region is crucial for understanding the physics behind these energetic events, and how they affect our planet, as the radiation emitted gets absorbed by Earth’s atmosphere. This can affect radio communication, GPS signals, and atmospheric drag on satellites. We typically use telescopes in space to observe the chromosphere, but seeing this elusive part of our nearest star with one’s own eyes during an eclipse leaves an everlasting impression. This photo was taken in Nebraska, USA in 2017.
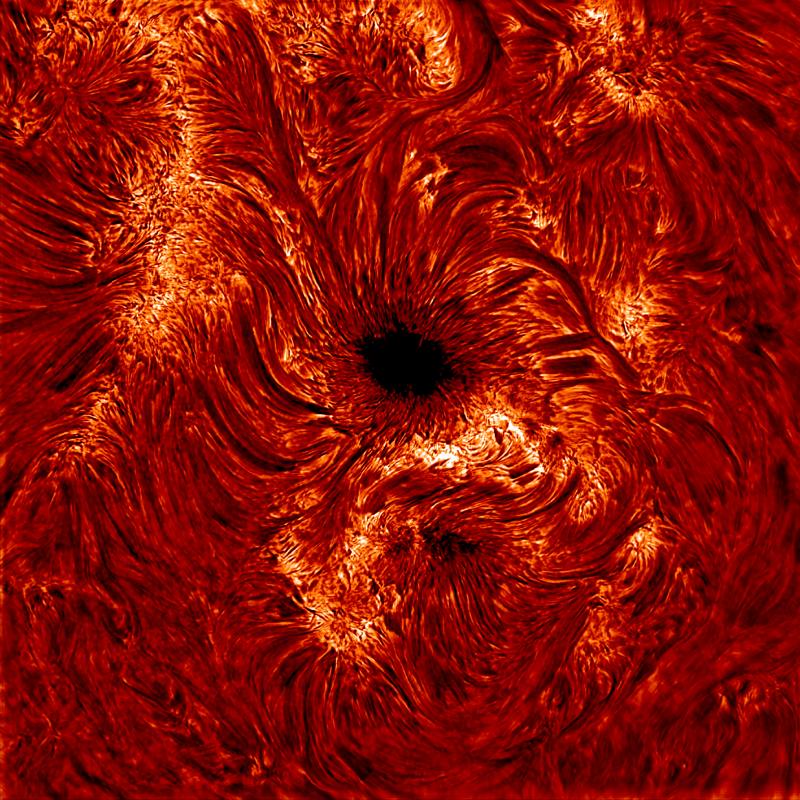
Drs. Samuel Grant & David Jess - Astrophysics Research Centre
From an observatory in the desert plains of New Mexico, USA, the dynamic atmosphere of our Sun is imaged with unparalleled contrast. One such image shows a dark region known as a ’sunspot’, a structure many times larger than the Earth, and the strongest magnet in the Solar System. The Sun provides an ideal laboratory for the study of how magnetic fields and hot plasma behave, to better understand how to harness them on Earth. For the images taken here, the focus is on solving a centuries old mystery, how the upper reaches of the solar atmosphere are 1 million degrees hotter than the solar surface, the ‘coronal heating paradox’. These sunspots may hold the key, in the forms of waves that that propagate throughout them, carrying heat throughout the atmosphere.
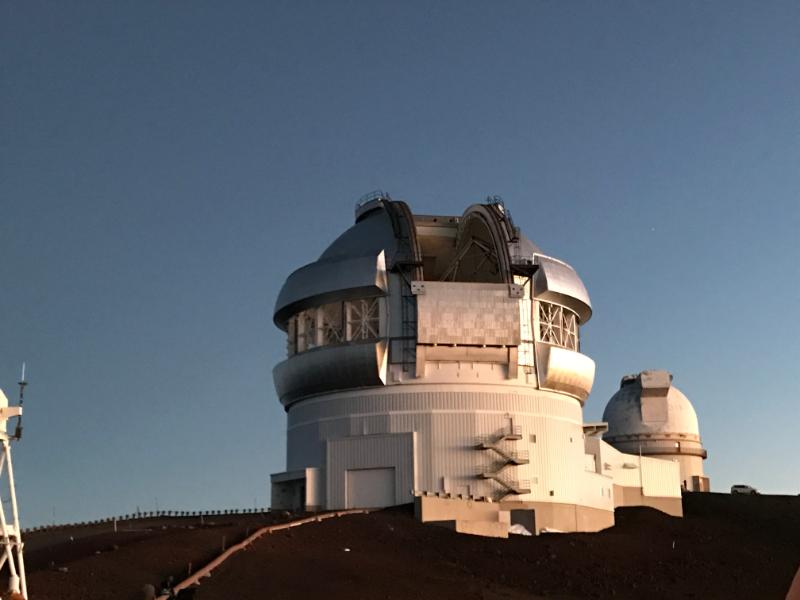
Dr Meg Schwamb - Astrophysics Research Centre
The 8.1-m Gemini Observatory (Gemini North) getting ready for an evening of observing. The telescope is located on Maunakea on the Big Island of Hawai'i. I use the telescope to study the reflected sunlight from distant Solar System objects, in order to learn what these small icy bodies can tell us about the formation and evolution of our Solar System.
Dr. Charlotte Palmer - Centre for Plasma Physics
In the summer, the buzz of bumble bees can be heard amongst the flowers. Many scientists study these bees and their intricate dances that are used to direct other bees to the location of the most succulent food sources, but how do they find them in the first place. As the bumble bees flap their wings, they build up charge, in the same way as rubbing a balloon on your hair. Like your hair, sticking to the balloon, pollen from the flowers then sticks to the bees more easily. This is because while the bees carry a small positive charge, the flowers are slightly negatively charged. After the bee visits the flower, the charge of the flower is neutralised for a while. Studies have now shown that the bees can sense whether the flower is still negatively charged or has already been neutralised and use this to make sure they visit flowers that still contain sweet, sweet nectar.
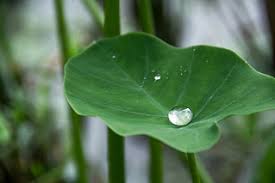
Musa 'Life is like a drop of water on a taro leaf' from Flickr
If plants need water, then why is this leaf repelling water? Turns out, in certain climates, the ability to quickly shed surface water provides a survival advantage to some plants. Surfaces that repel water, like the leaves of the Colocasia plant, are called hydrophobic. Microscopic textures play a huge role in hydrophobicity. For example, the leaves of the Colocasia plant are covered with waxy, microscopic bumps that prevent water drops from being able to stick, or adhere, to the leaf. (Imagine a Ping-Pong ball balancing on the teeth of a comb—that’s kind of how a water drop sitting on a Colocasia leaf would look if you were to zoom in really close). The tendency of liquid surfaces to shrink into the minimum surface area possible is calledSurface tension, an interesting property of liquid.As a bonus, the water drops collect dust and dirt as they roll along the leaf—in other words, the leaf surface is self-cleaning!
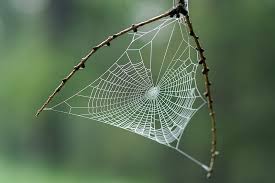
Rottonara 'Spiderweb' from Pixabay
Unlike Spider-Man, spiders make their own natural silk in their bodies via special glands found in their abdomen. Love them or hate them, but their innate ability to construct complicated pieces of natural architecture in the form of webs is nothing short of amazing. First, spider silk is five times stronger than steel - if human-size, it would be tough enough to snag a large aircraft. Scientists found that each strand, which is 1000 times thinner than a human hair, is actually made up of thousands of nano-strands (nanometre is a billionth of meter). Secondly, they use two different types of silks to make the web – a rigid type to construct the frame (spokes and outer supports of the web) and a stickier, more elastic silk for the radial parts (scaffolding). Hence no matter where you pull a single silk thread, it should break only where it’s pulled, instead of causing widespread damage.