Mining for Physics Gold
CQMT students use a new experimental technique to unveil unique topological structure in a ferroelectric material.
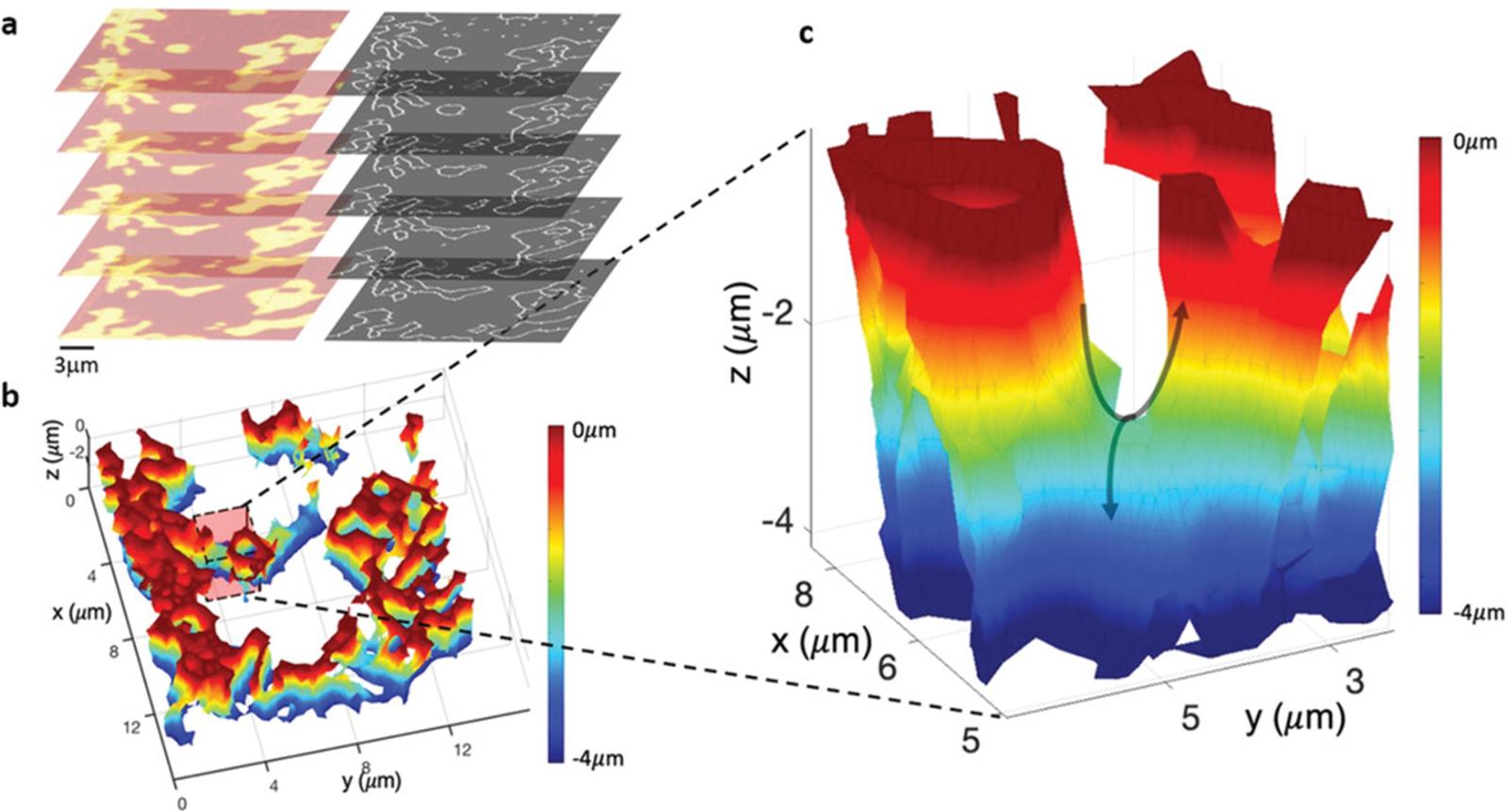
Experimental researchers in the Centre for Quantum Materials and Technology (CQMT) have worked alongside theorists to uncover details about the unique structure of an interesting material for the first time, by taking a relatively new technique a step further.
The applications and functionality of materials are continuously being explored in the search for future nanotechnologies. With this has come a surge of research directed towards a particular class of material known as ferroelectrics. These are materials which possess a spontaneous electric polarisation that can be reversed in direction by the application of an electric field. Regions of uniform polarisation within the material are known as domains, which are separated by a nanometre-scale interface known as a domain wall. Over the last few decades there has been a lot of excitement surrounding the prospect of using ferroelectric domains and domain walls for applications ranging from data storage to active components in mobile nanocircuitry.
More importantly for this work, ferroelectric materials can show profoundly interesting behaviour from the perspective of topology. In a general and somewhat simple sense, topology describes how the shape of an object can change by continuous deformation without any discrete jumps, while still maintaining its fundamental properties (for example, the number of holes it has). If one object can be transformed into another as a result of a smooth change, the two are topologically equivalent. In this sense, a coffee mug and a doughnut are the same: they both have one hole, and you can change from one to the other without any interruptions. To transform a coffee mug into a pretzel, however, requires another hole to be ripped, which is an abrupt (or ‘discontinuous’) change, making the two topologically distinct. The patterns formed within domain microstructures in ferroelectrics can lead to exotic topological features. High resolution techniques such as transmission electron microscopy have been used to study those on the scale of a few nanometres, such as ferroelectric vortices and skyrmions. However, these topological entities can be larger (on the scale of a few microns), which calls for new methods of experiment to study them.
Lead germanate is a uniaxial ferroelectric, which means the polarisation is restricted to one axis and can therefore only have two directions (up and down or left and right depending on the viewing direction). We have shown that the polarisation can rotate within the crystal, breaking the condition usually imposed by uniaxial ferroelectrics and forming a new topological entity. This has both changed the way in which we view the domain microstructure in the crystal and may go towards answering a more fundamental question that has bothered researchers for quite some time, about the domain walls in lead germanate.
A crucial method for studying domain microstructures in ferroelectrics is piezoresponse force microscopy; this is a technique which relies on the fact that all ferroelectrics are also piezoelectric. This means an applied voltage will result in a mechanical deformation of the material and vice versa. Applying this principle to the technique allows for domains with a specific orientation of polarisation to be distinguished from others. Until recently, information collected during PFM imaging has been exclusively two-dimensional. Researchers in CQMT (work led by PhD students Jesi Maguire and Conor McCluskey) have taken a relatively new technique a step further to access the third dimension of lead germanate, a ferroelectric material which was thought to host interesting topological features. This technique is tomographic PFM (TPFM), which takes conventional PFM a step further by applying significant forces to the metallic probe. This results in a removal of sequential layers of material, while simultaneously imaging the surface. The end result is a collection of images which represent the characteristic features of the domain structure throughout the depth of the material.
Figure 1: Domain wall saddle points in lead germanate revealed via tomographic piezoresponse force microscopy
The three-dimensional picture of lead germanate that we have created allows us to conclude two important things: the first is that the domain microstructure does not consist of isolated domains inside a matrix of another, as was initially thought, but instead the domain wall interestingly forms a saddle point at the position (see Figure 1) where the two domain types merge, a unique and interesting structure in itself. The second answers a more fundamental question about the behaviour at the domain walls. It has been well established that ferroelectric domain walls can often display enhanced conductivity in comparison to surrounding domains. This is surprisingly not true, however, for lead germanate. The interesting domain structure revealed by our TPFM experiments offers an explanation for this. The research findings have been recently published in Advanced Materials, one of the most highly regarded journals in physics and materials science.
The Queen’s University team worked in collaboration with teams from University of Picardie, Southern Federal University, Terra Quantum AG, City College of the City University of New York, JoẐef Stefan Institute, Norwegian University of Science and Technology and the University of Nebraska–Lincoln.